Timing Is Everything: Acoustic Niche Partitioning in Two Tropical Wet Forest Bird Communities
- Department of Biology, University of Hawai‘i at Hilo, Hilo, HI, United States
When acoustic signals sent from individuals overlap in frequency and time, acoustic interference and signal masking may occur. Under the acoustic niche hypothesis (ANH), signaling behavior has evolved to partition acoustic space and minimize overlap with other calling individuals through selection on signal structure and/or the sender’s ability to adjust the timing of signals. Alternately, under the acoustic clustering hypothesis, there is potential benefit to convergence and synchronization of the structural or temporal characteristics of signals in the avian community, and organisms produce signals that overlap more than would be expected by chance. Interactive communication networks may also occur, where species living together are more likely to have songs with convergent spectral and or temporal characteristics. In this study, we examine the fine-scale use of acoustic space in montane tropical wet forest bird communities in Costa Rica and Hawai‘i. At multiple recording stations in each community, we identified the species associated with each recorded signal, measured observed signal overlap, and used null models to generate random distributions of expected signal overlap. We then compared observed vs. expected signal overlap to test predictions of the acoustic niche and acoustic clustering hypotheses. We found a high degree of overlap in the signal characteristics (frequency range) of species in both Costa Rica and Hawai‘i, however, as predicted under ANH, species significantly reduced observed overlap relative to the random distribution through temporal partitioning. There was little support for acoustic clustering or the prediction of the network hypothesis that species segregate across the landscape based on the frequency range of their vocalizations. These findings constitute strong support that there is competition for acoustic space in these signaling communities, and this has resulted primarily in temporal partitioning of the soundscape.
Introduction
Acoustic signaling is a major form of social behavior in many terrestrial and aquatic organisms. When acoustic signals sent from individuals overlap in frequency and time, acoustic interference and signal masking occurs, which may reduce the receiver’s ability to discriminate information from the signal (Klump, 1996; Brumm and Slabbekoorn, 2005). Under the acoustic niche hypothesis (ANH; Krause, 1987, 1993), signaling behavior has evolved to minimize overlap with heterospecific calling individuals through selection on signal structure and the sender’s ability to adjust the timing of signals. This hypothesis may be viewed as an extension of the niche theory of Hutchinson (1957) whereby acoustic space is a resource that organisms may compete for and that can be partitioned both spectrally (frequency range of the signal) and temporally.
The ANH is central to describing how animal signals in diverse calling communities are dispersed in space and time and is a major organizing hypothesis in the field of soundscape ecology (Pijanowski et al., 2011). While the ANH makes intuitive sense and is based on both anecdotal and empirical evidence from a broad range of studies and signaling taxa (Farina and James, 2016), the degree to which organisms partition acoustic space in order to reduce interference remains the subject of recent debate (Tobias et al., 2014). Studies in biodiverse calling communities such as cicadas (Sueur, 2002), crickets (Schmidt et al., 2013), anurans (Chek et al., 2003; Sinsch et al., 2012; Villanueva-Rivera, 2014), and birds (Planqué and Slabbekoorn, 2008; Kirschel et al., 2009; Luther, 2009) have found evidence for niche partitioning through the apparent evolution of signal character displacement among species (e.g., by adjusting the frequency range of a signal to minimize overlap with those of other calling species in the community). However, acoustic signaling across a calling community typically occurs within a relatively narrow frequency range that reflects transmissibility of the signal in a particular habitat (Acoustic Adaptation Hypothesis; Morton, 1975; Ey and Fischer, 2009), body size and morphology of the sound producing organs (Ryan and Brenowitz, 1985; Suthers and Zollinger, 2008; Friis et al., 2021), the receiver’s ability to detect and interpret signals (Wiley and Richards, 1982), and sexual selection (Mikula et al., 2021). Thus, acoustic signals are relatively constrained in the way their spectral characteristics can vary to reduce interference with other biotic and abiotic sounds.
Time is a second major dimension along which acoustic niche partitioning may occur. Studies have demonstrated that birds can adjust the fine-scale timing of their signals (as opposed to adjusting the signals themselves) to take advantage of temporal gaps in acoustic space and thus minimize temporal acoustic overlap with other birds (Ficken et al., 1974; Popp et al., 1985; Suzuki et al., 2012; Yang et al., 2014) and even insects (Hart et al., 2015). However, those studies that have reported temporal adjustments in signals generally did so for select species or species groups and not the signaling community as a whole. Because the time axis of the acoustic niche is less constrained and thus may be more finely partitioned than the spectral axis, there is great potential for the evolution of behaviors that allow individuals within diverse calling assemblages to adjust the timing of their signals to avoid other signals at the community level. Thus, ANH may operate more through the temporal dimension than the spectral one.
Alternately, signals produced in diverse calling communities may converge in their spectral characteristics, timing, or both. Cody (1969, 1973) and Grether et al. (2009) described convergence in song and other behaviors in sympatric species that may function to reduce interspecific competition. Song characteristics may also converge on spectra that maximize the propagation of vocalizations under the characteristics of the habitat (Cardoso and Price, 2010), or they may converge in time due to intentional song overlapping (Todt and Naguib, 2000; Malavasi and Farina, 2013). Song within diverse signaling communities may also function as an extended communication network that selects for the clustering of species with similar signaling characteristics (Tobias et al., 2014). Under the acoustic clustering hypothesis, instead of partitioning acoustic space, organisms in diverse signaling communities produce signals that converge structurally and/or temporally and that overlap more than would be expected by chance. This hypothesis also predicts that species living together are more likely to have songs with convergent spectral and or temporal characteristics than those not living together (Tobias et al., 2014).
Tropical wet forests have the most species-rich assemblages of organisms that signal acoustically, and thus competition for acoustic niche-space is expected to be strongest there (Slabbekoorn, 2004; Planqué and Slabbekoorn, 2008). In this study, we examine the fine-scale timing of signals in acoustic space and the relevance of the acoustic niche and acoustic clustering hypotheses in montane tropical wet forest bird communities in both Hawai‘i and Costa Rica. We first assessed the potential for acoustic interference by characterizing the frequency range of signals produced by bird species we recorded during the dawn chorus in each community. We then identified the species associated with each recorded signal at multiple recording stations in each community and measured observed signal overlap within and among species. Null models (Gotelli and Graves, 1996; Masco et al., 2015) were used to generate random distributions of expected signal overlap, and observed vs. expected signal overlap was compared to test predictions of the acoustic niche and acoustic clustering hypotheses for the two bird communities. We also used null models to test a prediction of the acoustic clustering hypothesis that species living together are more likely to have songs with convergent characteristics.
Materials and Methods
Study Sites
The Costa Rica study site was within an approximately 360 ha forest fragment at the Organization for Tropical Studies (OTS) Las Cruces Biological Field Station in southern Costa Rica at elevations between 1,025 and 1,200 m. This site is dominated by a mix of primary and secondary wet forest with a canopy up to 30 m tall and a midcanopy layer comprised of broadleaf trees, palms, and tree-ferns. Mean annual precipitation ranges from 3,500 to 4,000 mm and mean annual temperature at Las Cruces Biological Station is ∼21°C (Zahawi et al., 2015). The bird checklist maintained by OTS for the station is comprised of over 400 species, including many migrants (Martìnez, 2010). The Hawai‘i study site was within the Maulua tract of Hakalau Forest National Wildlife Refuge on the island of Hawai‘i. The canopy at this site is dominated by Metrosideros polymorpha-Acacia koa trees up to 25 m tall with a mid-canopy of at least six native tree and tree-fern species. Mean annual precipitation is approximately 2,250 mm and mean annual daily temperature is ∼15°C at the study site (Juvik and Juvik, 1998). Hakalau contains the most intact forest bird community remaining in the state with nine native species, plus an additional four non-native species that are common in the forest. Within each of the two study sites, we recorded bird songs using autonomous acoustic recorders (SM2 Wildlife Acoustics Inc.) placed ∼1 meter above the ground at six different locations separated by at least 200 m. Recordings were made for 3–5 days at each location, depending on weather conditions. We programmed the acoustic recorders to record at 5-min intervals (5-min on and 5-min off) from first light until 11:30 during the breeding season months of June through July 2012 in Costa Rica and March through April 2015 in Hawai‘i. Recordings were made in WAV file format at a sampling rate of 44.1 kHz using a single omnidirectional microphone (SMX-II Wildlife Acoustics) with a sensitivity of −35 dBV/pa and frequency response of 20–20,000 Hz. This study relied solely on the use of passive acoustic monitors placed in the forest to collect acoustic data. No animals were captured, handled, housed, monitored, or followed. No University IACUC permits were required for this study. Permission to conduct fieldwork was given by landowners in both Costa Rica (Organization for Tropical Studies) and Hawai‘i (Hakalau Forest National Wildlife Refuge).
Acoustic and Statistical Analysis
Within each study site, we randomly selected 1 day at each of the six sensor locations. We then selected three consecutive 5-min recordings per day during the dawn chorus (when birds are most acoustically active) that did not contain rain for acoustic analysis. Cicada choruses, particularly those of the large-bodied cicada (Zammara smaragdina), generally occur near the end of the dawn chorus and can alter signal production for birds in Costa Rica [22]. We excluded recordings with cicada choruses from the analyses, resulting in two recording locations in Costa Rica with only two 5-min recordings available. All selected recordings were then visualized on a spectrogram using Raven Pro 1.5 software (K. Lisa Yang Center for Conservation Bioacoustics, 2014).
We identified the bird species associated with each vocalization, then used the selection tool in RavenPro 1.5 to determine the minimum and maximum frequency and signal start and end time for all bird vocalizations within each 5-min recording period. Only selected vocalizations > 5 dB of the background noise of each recording were included in the analysis. All signals were measured using a Hann window type and a window size of 23.2 ms, window overlap of 50%, and DFT (discreet Fourier transform) size of 1024 samples. For each of the six recording locations in Costa Rica and Hawai‘i, we calculated total species richness across the 10–15 min recording periods, and vocalization rate as the total number of vocalizations within a 5-min time period. We examined differences in frequency range (Hz), signal length (s), vocalization rate (per 5-min period), and species richness between Hawai‘i and Costa Rica using Wilcoxon Rank Sum tests in R (R Core Development Team, 2018, version 3.5.1).
We used a novel null model approach to test the ANH. For each of the six recording locations in Costa Rica and Hawai‘i (10–15-min recording period), we computed the observed number of overlapping pairs of vocalizations as the number of times any two vocalizations overlapped simultaneously on the temporal and spectral axes by at least 1-Hz. We then generated an expected level of overlap by randomizing the beginning of each vocalization (within each 10–15-min recording period) but keeping the duration and frequency range unchanged. This was repeated 500 times to generate an expected distribution of vocalization overlaps. We then used the pnorm R function to calculate P-values as the probabilities of getting the observed numbers of overlapping pairs of vocalizations, or more extreme numbers, if the null hypothesis is true. Significantly fewer (P-value < 0.025) observed overlaps than expected based on the null distribution supports the ANH, while significantly more observed overlaps than expected (P-value > 0.975) supports the acoustic clustering hypothesis.
To test an additional prediction of the acoustic clustering hypothesis that species with similar vocalization characteristics are more likely to co-occur across the landscape, we calculated the observed number of species pairs with vocalizations that overlapped in mean frequency range at each recording location (six in Costa Rica and six in Hawai‘i). We then calculated an expected number of species pairs with overlapping vocalizations by randomizing the occurrence of species at each recording location (from the pool of all species recorded in either Costa Rica or Hawai‘i) while keeping species richness at that location unchanged. This was repeated 500 times to generate an expected distribution of number of species pairs that overlap in frequency and used the pnorm function in R to calculate P-values as described above. This allowed us to examine if the species detected at a recording location converge in their song characteristics more than those collected randomly from the pool of species that were detected from the study site.
Results
Vocalizations Pool
We detected 39 vocalizing species from Costa Rica and 10 from Hawai‘i in the recordings selected for analysis. Three of the 10 species from Hawai‘i were non-native (Cardinalis cardinalis, Leiothrix lutea, Zosterops japonicus) and accounted for < 10% of total vocalizations. There was a total of 2,880 vocalizations with a duration of 1,614 s from Costa Rica and 4,489 vocalizations detected with a duration of 6,097 s from Hawai‘i. According to species accumulation curves the recording length (10–15 min) was long enough to capture species diversity at a location (Figure 1). In all recording locations except one in Costa Rica (CR1), the number of recorded species reached a plateau by the end of the recording period.
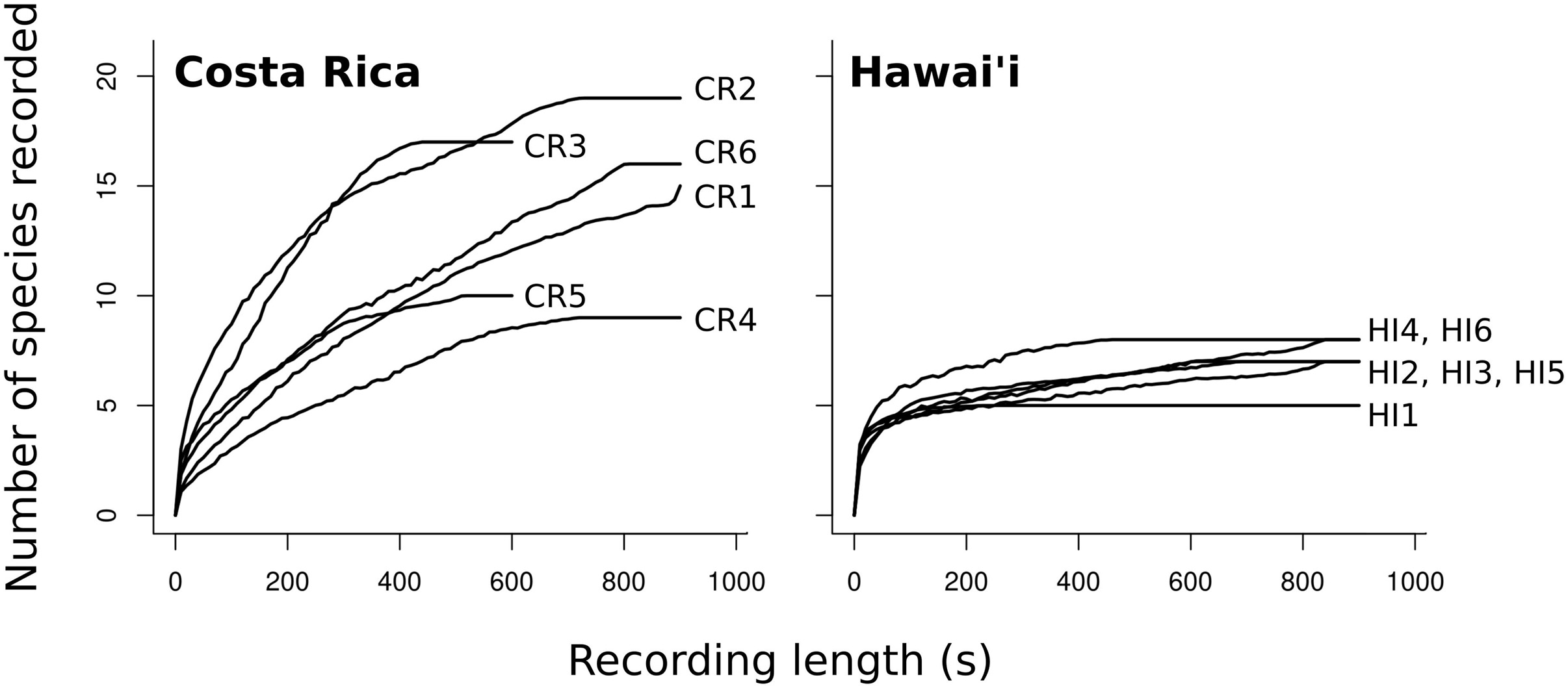
Figure 1. Species accumulation curves (mean of 500 iterations) for each of six recording locations in Costa Rica and Hawai‘i.
Potential for Overlap Between Species Vocalizations
We found a high degree of overlap in the frequency range used by species in both Costa Rica and Hawai‘i (Figure 2). In Hawai‘i, 44 of the 45 pairs of species (i.e., 98%) had potentially overlapping vocalizations (i.e., the mean frequency range of their signals overlapped by at least 1-Hz). Indeed, only the native Loxops coccineus and the non-native Cardinalis cardinalis vocalized using totally different frequencies (Figure 2). In Costa Rica, 570 of the 741 pairs of species (i.e., 77%) had potentially overlapping vocalizations. The mean frequency range used by species was significantly higher in Hawai‘i than in Costa Rica (Figure 3A, P = 0.0014) while species’ vocalization lengths were similar (Figure 3B, P = 0.1320). Vocalization rates (i.e., the number of recorded vocalizations per 5-min. period) tended to be lower in Costa Rica (median = 135) than Hawai‘i (median = 258) (Figure 3C, P = 0.1320), but the number of species per location was significantly higher in Costa Rica (median = 16) than in Hawai‘i (median = 7) (Figure 3D, P = 0.0047).
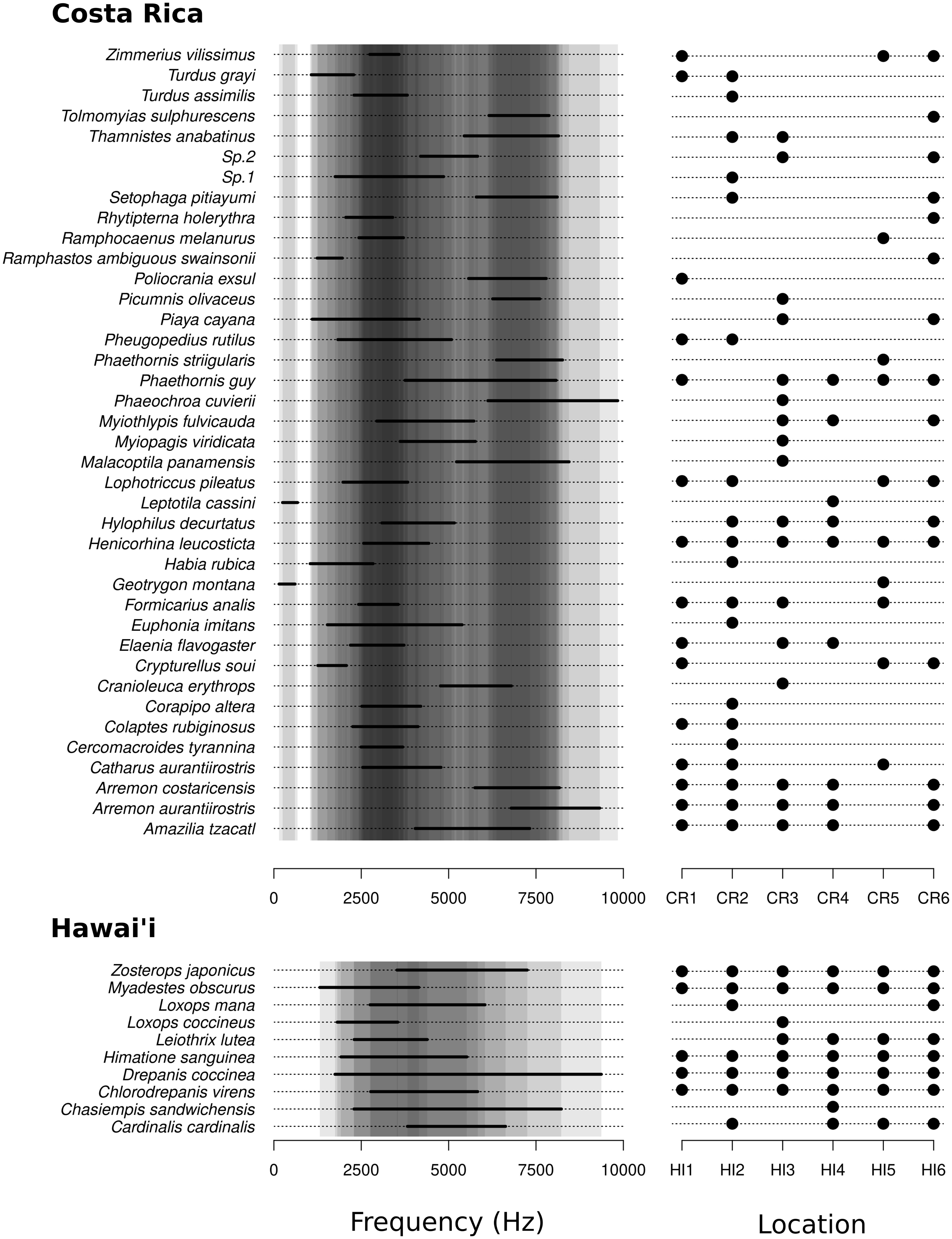
Figure 2. List of detected species with their vocalization ranges (left figures) and their locations of occurrence (right figures) in Costa Rica and Hawai‘i. On the left figures, gray background represents potential overlapping in a given frequency, the darker the background, the higher the number of species using this frequency.
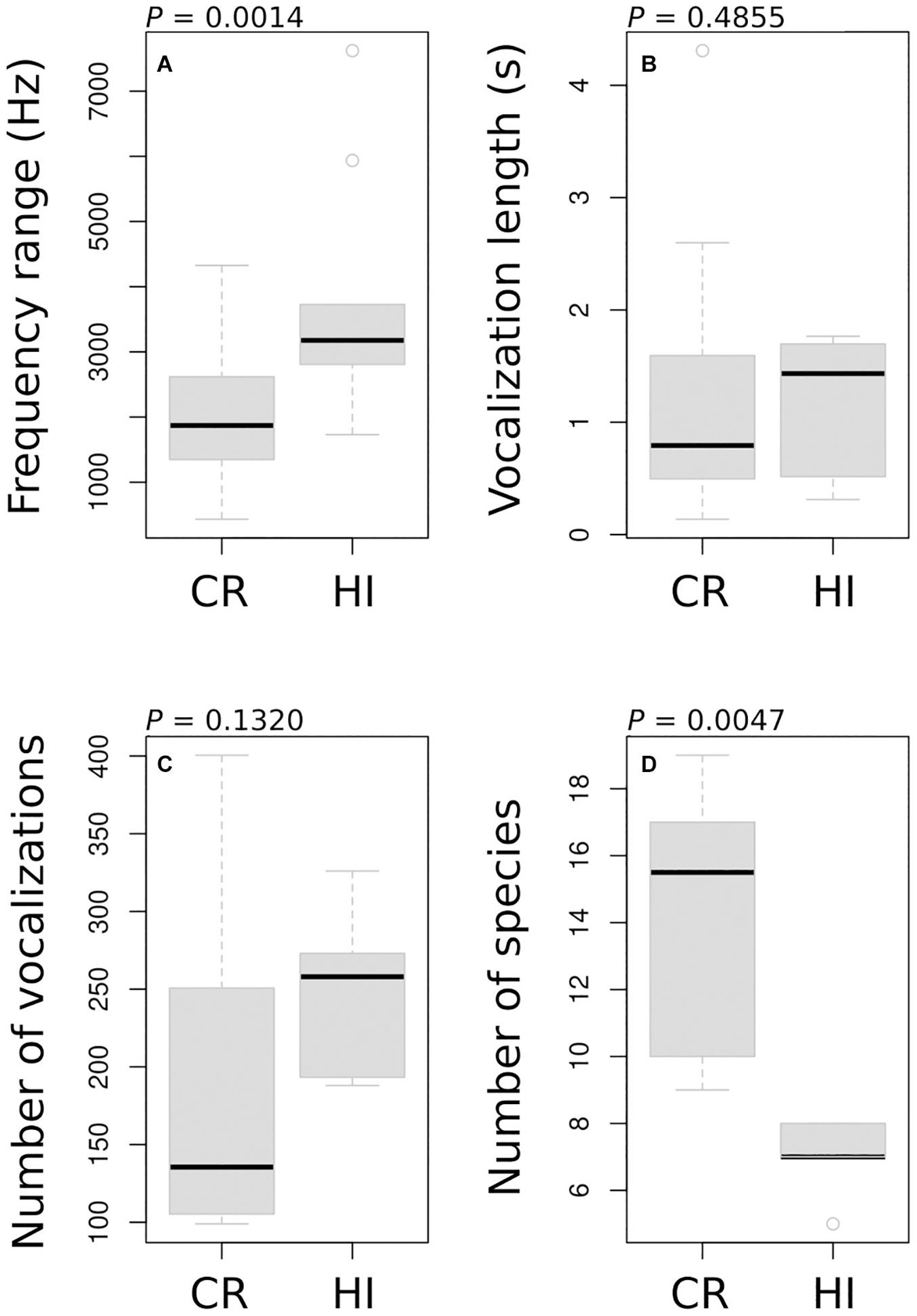
Figure 3. Comparison of species vocalization characteristics (A,B), the number of vocalizations per 5-min record (C), and number of species recorded per location (D) between Costa Rica (CR) and Hawai‘i (HI) (P-value indicates the significance of Wilcoxon’s rank sum test).
Temporal Overlap
Observed signal overlap was far less than expected if the beginning time of each vocalization was random. Despite the high degree of overlap in frequency range used by species as described above for Costa Rica and Hawaii, the temporal distribution of vocalizations was arranged in a way to reduce vocalization overlaps (Figure 4). In most recording locations the observed number of pairs of overlapping vocalizations (in both time and frequency) was significantly lower than expected under random temporal distribution of vocalizations.
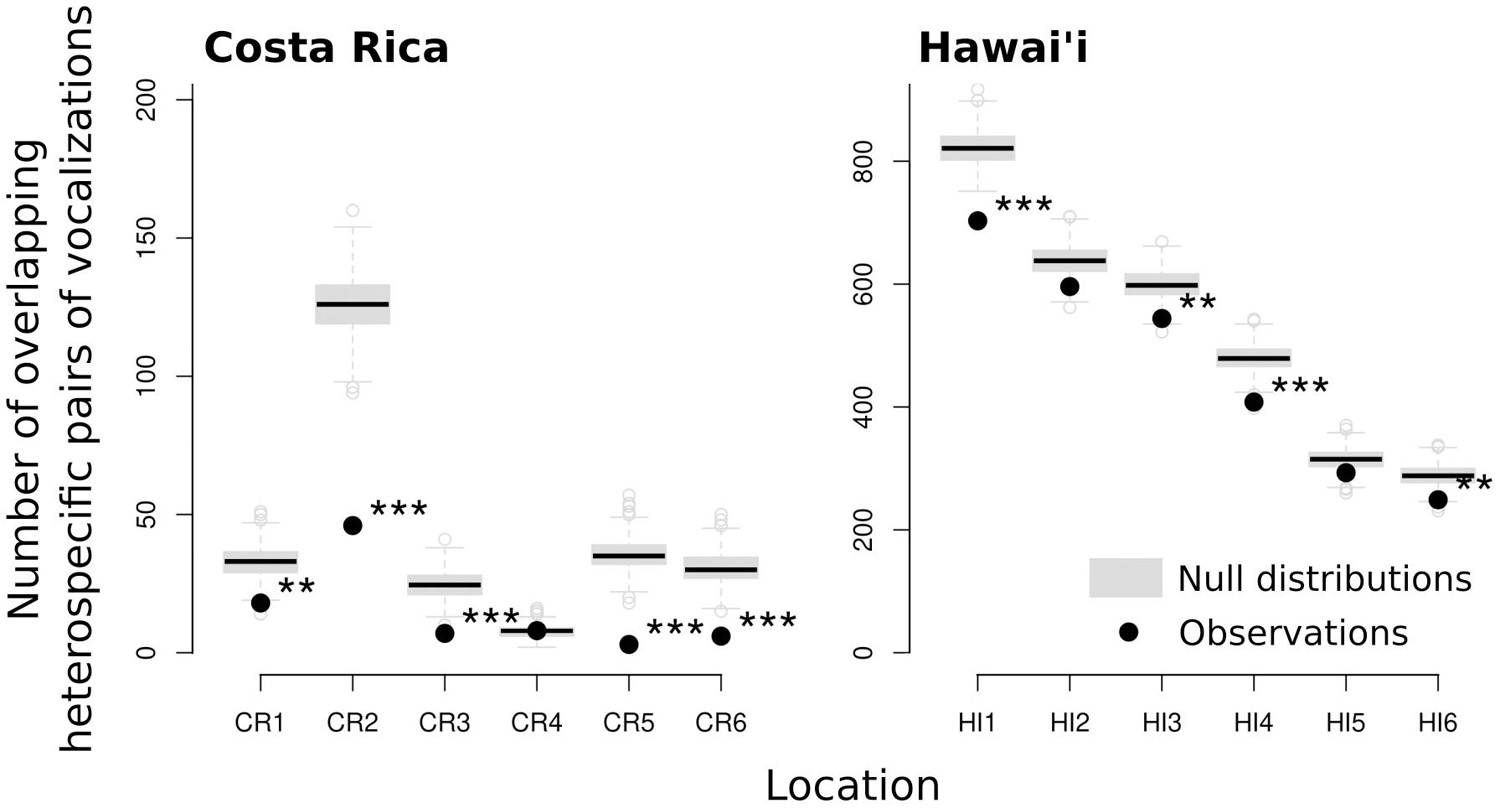
Figure 4. Comparison of observed and expected number of heterospecific pairs of vocalizations with overlapping vocalization times and frequencies in each location (significance “***”α = 0.001, “**”α = 0.01). The null distributions were generated using 500 randomizations of the beginning of vocalizations (keeping number, length and spectral characteristics unchanged).
Species Co-occurrence
There was little relationship between species distribution (recording locations the species was detected at) and the vocalization frequencies of species across recording locations in Costa Rica and Hawai‘i (Figure 5). In most locations the observed number of species pairs vocalizing in overlapping frequencies (by at least 1-Hz) did not differ significantly from expectations under random distribution of species between locations. However, in two locations in Costa Rica the observed number of species pairs vocalizing in overlapping frequencies was significantly higher than expected, indicating that species with overlapping frequencies were more likely to occur in these locations than species with non-overlapping frequencies.
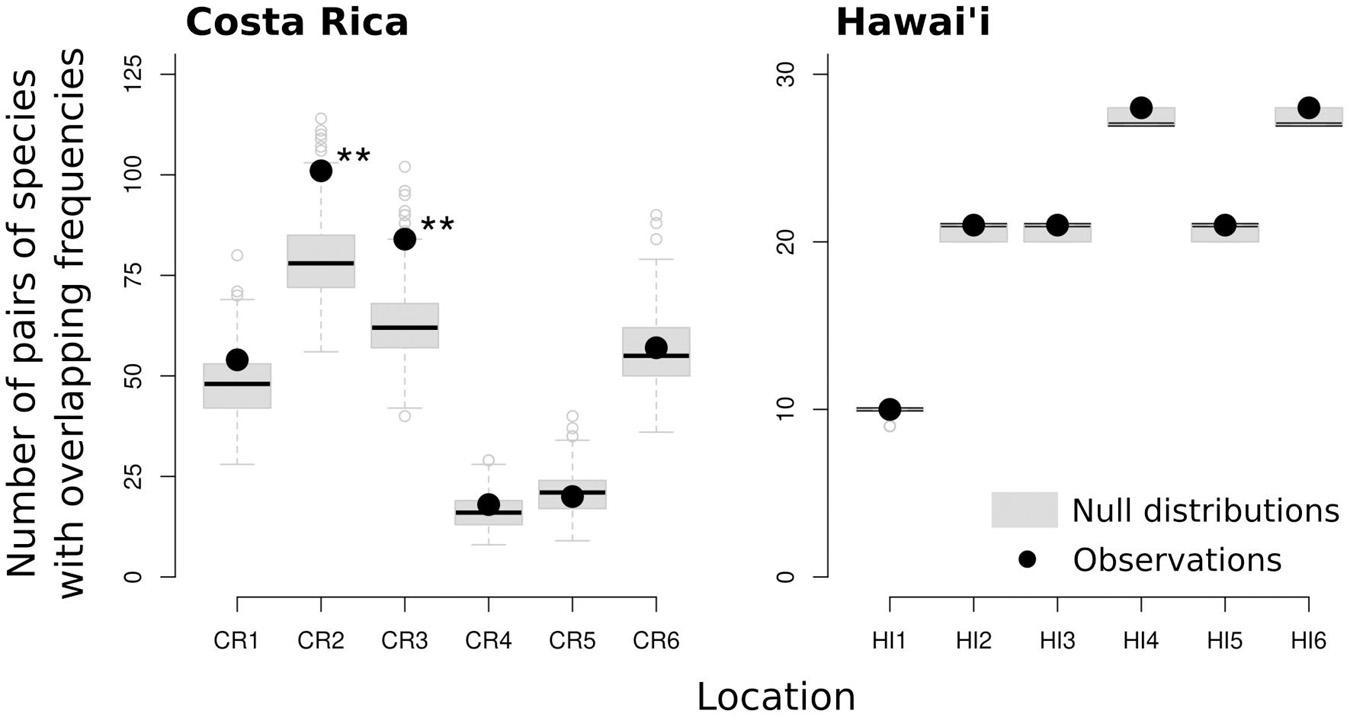
Figure 5. Comparison of observed and expected number of pairs of species with overlapping vocalization frequencies in each location (significance “**”α = 0.01). The null distributions were generated using 500 randomizations of species distribution across locations (keeping species richness per location unchanged).
Discussion
There was a high degree of overlap in the spectral characteristics of the signals (vocalization frequency range) of species in both Costa Rica and Hawai‘i, and thus great potential for interference between the different signals. However, we found significantly fewer observed overlaps of acoustic signals than expected if the beginning of signals were dispersed randomly at five out of six locations in Costa Rica and 4 out of six in Hawai‘i (Figure 4). We found only weak evidence for the prediction of the acoustic clustering hypothesis that species living together (at the level of recording location within a study site) are more likely to have songs with convergent spectral and or temporal characteristics than those not living together (Figure 5). There was also no support for the hypothesis that signals would be temporally clustered in acoustic space as there were no cases from our recording locations in either Costa Rica or Hawai‘i where the observed number of overlaps between signals was greater than expected (based on randomization of signal timing). Together, these findings indicate that birds adjust the timing of their signals to minimize spectral overlap at the community level and constitute strong support for ANH.
Adaptive timing of signal production has been demonstrated for select pairs or groups of species, but has not yet been shown to operate across entire bird communities within the short temporal scales as described here. Cody and Brown (1969) were the first to demonstrate that birds can reduce competition for acoustic space by adjusting the fine-scale timing of their signals. In this case wren-tits (Chamaea fasciata) and Bewick’s wrens (Thryomanes bewickii) were shown to sing asynchronously, based on when the other species was vocalizing. Similarly, Ficken et al. (1974) showed that two species of forest birds, the least flycatcher (Empidonax minimus) and red-eyed vireo (Vireo olivaceous), adjust the temporal pattern of their singing to reduce overlap among their songs. Popp et al. (1985) showed that four species that commonly sang in a Wisconsin broad-leaf forest, wood thrush (Hylocichla mustelina), eastern wood pewee (Contopus virens), great crested flycatcher (Myiarchus crinitus), and ovenbird (Seiurus aurocapilla), significantly avoided singing during the song of the other species. Yang et al. (2014) experimentally demonstrated that Eurasian wrens (Troglodytes troglodytes) avoid song overlaps by timing their songs to start right after the end of a stimulus song. Planqué and Slabbekoorn (2008) examined signal dispersion in a diverse Peruvian bird assemblage and found significantly less temporal overlap among select species pairs that vocalize in overlapping frequencies. Similarly, Luther (2009) created principal components from the acoustic characteristics of signals of 82 species in a Brazilian wet forest and found evidence of acoustic partitioning for those species that sing at similar locations and time. In particular, species that sang at a recording location during a 30-min period beginning at sunrise had songs that were more greatly dispersed in acoustic space than those that sang at different times throughout the morning. We should note that analysis of principal components of signal characteristics is a helpful way to examine how the signals of different species are dispersed relative to each other but cannot clarify differences in observed vs. expected (random) overlap of those characteristics in real time.
Can birds process incoming auditory signals quickly enough to avoid overlap between their own signals and those of others in a species rich calling community? The mechanisms by which birds are able to temporally partition acoustic space have received little attention, but center on how long it takes for a signal to travel from sender to receiver, and how long it takes the receiver to process it and make a decision to not sing. Sound travels approximately 1 m in 2.9 ms (0.0029 s) so it would take a signal at most about 90 ms to reach a receiver within its approximate 30 m radius signal envelope. Reaction times to auditory signals have been described for a range of species and environmental conditions, particularly for those that engage in elaborate duets that involve precise timing of signals among mates. Thorpe (1963) measured a mean reaction time of 144 ± 12.6 ms between first and second note in antiphonal duetting black-headed gonolek (Laniarius erythrogaster) pairs, and Power (1966) reported mean reaction times ranging from 100 to 164 ms in duetting orange-chinned parakeets (Brotogeris jugularis). Mean reaction time to sound stimuli for starlings (Sturnus vulgaris) in a laboratory setting was 80 ms ± 14.4 ms (Pomeroy and Heppner, 1977) while Cuthbert and Mennill (2007) described mean gaps between M and F phrases in Plain wren (Thryothorus modestus modestus) duets to be as little as 50 ms. Thus, birds may make a decision in as little as 50 ms or less to hold off on vocalizing if they detect another vocalization in very close proximity, with the lag time in the decision to not produce a vocal signal due to the presence of another signal increasing by 2.9 ms for each meter further away a signaler is located. The ability to avoid signal overlap would therefore increase with decreasing distance from signaler to receiver. This may be one reason (along with amplitude differences) why signals that are produced at greater distances overlap more than those produced nearby.
Birds may also make use of temporal patterns in song among acoustically active species, allowing them to better predict when empty “niches” will occur in acoustic space and to quickly fill them. Of course, timing songs based on the temporal patterns of other species can become complicated in a diverse calling community, and most studies of song timing based on the calls of other individuals have primarily been demonstrated for intraspecific song (Todt and Naguib, 2000). For example, both blackbirds (Wolffgramm and Todt, 1982) and nightingales (Hultsch and Todt, 1982) have been shown to base the timing of their song on the patterns of other calling individuals. In a diverse neotropical wet forest in Brazil, Luther (2008) demonstrated that four co-occurring species of birds coordinated both the timing and response of signals in order to reduce acoustic interference during the dawn chorus. Malavasi and Farina (2013) found evidence of coordinated choruses among different bird species in an Italian bird community whereby deliberate temporal overlaps occurred while at the same time avoiding spectral overlap and thus signal jamming. Some bird species may also be specialized for calling at different periods during the dawn chorus, possibly to take advantage of favorable environmental conditions for sound propagation. There may be a range of strategies among species in each community for avoiding overlap, and none are mutually exclusive. In fact, some of the acoustic overlaps we detected may be intentional forms of song-overlap within and among species (Todt and Naguib, 2000).
In weak support of the network hypothesis, we did observe significantly more species pairs with overlapping frequencies co-occurring than expected under the null at two out of six recording locations in Costa Rica, though there were none in Hawai‘i. This indicates that bird species in these forests may segregate to some degree across the landscape based on the frequency range of their vocalizations. It would be worthwhile to expand the number of sites and recording locations in Costa Rica to further explore the possibility that there is synchronization in the use of acoustic space through interactive communication networks. In contrast to our findings, and in support of the network hypothesis, Tobias et al. (2014) and Kleyn et al. (2021) reported significant clustering of acoustic signals in a species-rich wet forest bird community in southeast Peru and southeastern Brazil, respectively. However, the temporal scale at which clustering occurred was within 10-min time blocks, much larger than the finer scale of individual songs that we report here. The more subtle form of partitioning that we describe provides evidence that birds have evolved and/or learned behaviors to significantly reduce interference and masking by other birds signals primarily through fine-scale temporal partitioning. This reduces the selection pressure for the evolution of structurally dissimilar signals, and allows birds to produce signals that are better adapted for information transmission (e.g., mate attraction, territoriality, contact) in their particular habitat. This form of acoustic niche partitioning can occur even where signal synchrony and communication networks are common in the community. Thus, temporal acoustic niche partitioning and acoustic clustering (that results from communication networks) are not necessarily mutually exclusive, at least at the level at which they have been examined to date.
While we found evidence for acoustic niche partitioning in both communities, and despite similarities in signaling rate and in the range of spectral bandwidths used, we found that overlap between different vocalizations was more common in Hawai‘i than in Costa Rica (Figure 4), possibly because greater species richness in Costa Rica has led to stronger selection for reducing interspecific signal overlap there. In addition, the island of Hawai‘i is geologically younger than much of Costa Rica (∼0.5 vs. 2.5–15 my) (Alvarado and Cárdenes, 2016) so there are likely differences in length of time available for the evolution of signal character displacement between locations. There may be a phylogenetic effect as well—the Costa Rican bird community represents a far greater diversity of avian lineages than Hawai‘i, where most of the current species have evolved through adaptive radiation from just a handful of colonizations from distant continents (Freed et al., 1987; Lerner et al., 2011). Differences in signal function may also partially explain the different levels of signal overlap between the two communities. Most of the Costa Rica species sampled here maintain year-round territories (Stiles and Skutch, 1989), whereas most of the Hawaiian species are generally non-territorial outside the breeding season. The function of song may be more associated with promoting social cohesion, with less selection against reducing interspecific signal overlap, in communities with higher densities of individual species that are non-territorial.
Data Availability Statement
The datasets presented in this study can be found in online repositories. The names of the repository/repositories and accession number(s) can be found below: https://datadryad.org/stash/dataset/doi:10.5061/dryad.w6m905qkp.
Ethics Statement
Ethical review and approval was not required for the animal study because the study relied solely on the use of passive acoustic monitors placed in the forest to collect acoustic data. No animals were captured, handled, housed, monitored, or followed.
Author Contributions
PH and ES-G conceived the idea. PH acquired the data. TI, KP, ES-G, GT, and AT-J analyzed the data. PH, KP, and TI wrote the first draft. All authors contributed to interpreting the results and writing the final draft.
Funding
Financial support was provided through National Science Foundation (NSF) CREST awards (0833211 and 1345247) to PH.
Conflict of Interest
The authors declare that the research was conducted in the absence of any commercial or financial relationships that could be construed as a potential conflict of interest.
Publisher’s Note
All claims expressed in this article are solely those of the authors and do not necessarily represent those of their affiliated organizations, or those of the publisher, the editors and the reviewers. Any product that may be evaluated in this article, or claim that may be made by its manufacturer, is not guaranteed or endorsed by the publisher.
Acknowledgments
We thank the Organization for Tropical Studies (OTS) NAPIRE (Native American and Pacific Islander Research Experience) program and the staff of the OTS Las Cruces Biological Field Station, particularly Zak Zahawi. Michael Atencio-Picado also assisted with the identification of the song of some Costa Rican bird species. We also thank refuge managers and biologists at Hakalau Forest National Wildlife Refuge, including J. Krauss, S. Kendall, and T. Cady for providing permits, support, and study site access.
References
Alvarado, G. E., and Cárdenes, G. (2016). “Geology, Tectonics, and Geomorphology of Costa Rica: A Natural History Approach,” in Costa Rican Ecosystems, ed. M. Kappelle (Chicago: The University of Chicago Press), 30–63. doi: 10.7208/chicago/9780226121642.003.0003
Brumm, H., and Slabbekoorn, H. (2005). Acoustic communication in noise. Adv. Stud. Anim. Behav. 35, 151–209.
Cardoso, G., and Price, T. (2010). Community convergence in bird song. Evol. Ecol. 24, 447–461. doi: 10.1007/s10682-009-9317-1
Chek, A. A., Bogart, J. P., and Lougheed, S. C. (2003). Mating signal partitioning in multi-species assemblages: a null model test using frogs. Ecol. Lett. 6, 235–247. doi: 10.1046/j.1461-0248.2003.00420.x
Cody, M. L. (1969). Convergent characteristics in sympatric species- a possible relation to interspecific competition and aggression. Condor 71, 223–239. doi: 10.2307/1366300
Cody, M. L., and Brown, J. H. (1969). Song asynchrony in neighboring bird species. Nature 222, 778–780. doi: 10.1038/222778b0
Cuthbert, J. L., and Mennill, D. J. (2007). The duetting behavior of Pacific coast plain wrens. Condor 109, 686–692. doi: 10.1650/8234.1
Ey, E., and Fischer, J. (2009). The “acoustic adaptation hypothesis” – a review of the evidence from birds, anurans and mammals. Bioacoustics 19, 21–48. doi: 10.1080/09524622.2009.9753613
Farina, A., and James, P. (2016). The acoustic communities: definition, description, and ecological role. Biosystems 147, 11–20. doi: 10.1016/j.biosystems.2016.05.011
Ficken, R. W., Ficken, M. S., and Hailman, J. P. (1974). Temporal pattern shifts to avoid acoustic interference in singing birds. Science 183, 762–763. doi: 10.1126/science.183.4126.762
Freed, L. A., Conant, S., and Fleischer, R. C. (1987). Evolutionary ecology and radiation of Hawaiian passerine birds. Trends Ecol. Evol. 2, 196–203. doi: 10.1016/0169-5347(87)90020-6
Friis, J. I., Dabelsteen, D., and Cardoso, G. C. (2021). Contingency and determinism in the evolution of bird song sound frequency. Sci. Rep. 11:11600.
Gotelli, N. J., and Graves, G. R. (1996). Null models in Ecology. Washington: Smithsonian Institute Press.
Grether, G. F., Losin, N., Anderson, C. N., and Okamoto, K. (2009). The role of interspecific interference competition in character displacement and the evolution of competitor recognition. Biol. Rev. 84, 617–635. doi: 10.1111/j.1469-185x.2009.00089.x
Hart, P. J., Hall, R., Ray, W., Beck, A., and Zook, J. (2015). Cicadas impact bird communication in a noisy tropical rainforest. Behav. Ecol. 26, 839–842. doi: 10.1093/beheco/arv018
Hultsch, H., and Todt, D. (1982). Temporal performance roles during vocal interactions in nightingales (Luscinia megarhynchos). Behav. Ecol. Sociobiol. 11, 253–260. doi: 10.1007/bf00299302
Juvik, S. P., and Juvik, J. O. (eds) (1998). Atlas of Hawai‘i, third edition. Hawaii: University of Hawai‘i Press.
K. Lisa Yang Center for Conservation Bioacoustics (2014). Raven Pro: Interactive Sound Analysis Software (Version 1.5) [Computer Software]. Ithaca, NY: The Cornell Lab of Ornithology. Available online at: http://ravensoundsoftware.com/
Kirschel, A. N. G., Blumstein, D. T., Cohen, R. E., Buermann, W., Smith, T. B., and Slabbekoorn, H. (2009). Birdsong tuned to the environment: green hylia song varies with elevation, tree cover, and noise. Behav. Ecol. 20, 1089–1095. doi: 10.1093/beheco/arp101
Kleyn, T., da Cruz Kaizer, M., and Passos, L. F. (2021). Sharing sound: avian acoustic niches in the Brazilian Atlantic Forest. Biotropica 53, 658–670. doi: 10.1111/btp.12907
Klump, G. M. (1996). “Bird communication in the noisy world,” in Ecology and Evolution of Acoustic Communication in Birds, eds D. E. Kroodsma and E. H. Miller (New York: Cornell Univ. Press).
Krause, B. L. (1987). Bioacoustics, Habitat Ambience in Ecological Balance. Whole Earth Rev. 57, 14–18.
Lerner, H. R., Meyer, M., James, H. F., Hofreiter, M., and Fleischer, R. C. (2011). Multilocus resolution of phylogeny and timescale in the extant adaptive radiation of Hawaiian honeycreepers. Curr. Biol. 21, 1838–1844. doi: 10.1016/j.cub.2011.09.039
Luther, D. (2008). Signaller:Receiver coordination and the timing of communication in Amazonian birds. Biol. Lett. 4, 651–654. doi: 10.1098/rsbl.2008.0406
Luther, D. (2009). The influence of the acoustic community on songs of birds in a Neotropical rain forest. Behav. Ecol. 20, 864–871. doi: 10.1093/beheco/arp074
Malavasi, R., and Farina, A. (2013). Neighbors’ talk: interspecific choruses among songbirds. Bioacoustics 22, 33–48. doi: 10.1080/09524622.2012.710395
Martìnez, D. (2010). Lista de aves de la Estacioìn Bioloìgica Las Cruces y alrededores. Available online at: http://www.ots.ac.cr
Masco, C., Allesina, S., Mennill, D. J., and Pruett-Jones, S. (2015). The song overlap null model generator (SONG): a new tool for distinguishing between random and non-random song overlap. Bioacoustics 25, 29–40. doi: 10.1080/09524622.2015.1079734
Mikula, P., Valcu, M., Brumm, H., Bulla, M., Forstmeier, W., Petrusková, T., et al. (2021). A global analysis of song frequency in passerines provides no support for the acoustic adaptation hypothesis but suggests a role for sexual selection. Ecol. Lett. 24, 477–486. doi: 10.1111/ele.13662
Morton, E. S. (1975). Ecological sources of selection on avian sounds. Am. Nat. 109, 17–34. doi: 10.1086/282971
Pijanowski, B. C., Villanueva-Rivera, L. J., Dumyahn, S. L., Farina, A., Krause, B. L., Napoletano, B. M., et al. (2011). Soundscape Ecology: the Science of Sound in the Landscape. BioScience 61, 203–216.
Planqué, R., and Slabbekoorn, H. (2008). Spectral overlap in songs and temporal avoidance in a Peruvian bird assemblage. Ethology 114, 262–271. doi: 10.1111/j.1439-0310.2007.01461.x
Pomeroy, H., and Heppner, F. (1977). Laboratory determination of startle reaction time of the starling (Sturnus vulgaris). Anim. Behav. 25, 720–725. doi: 10.1016/0003-3472(77)90121-x
Popp, J. W., Ficken, R. W., and Reinartz, J. A. (1985). Short-term temporal avoidance of interspecific acoustic interference among forest birds. Auk 102, 744–748.
Power, D. M. (1966). Antiphonal dueting and evidence for auditory reaction time in the Orange-chinned Parakeet. Auk 83, 314–319. doi: 10.2307/4083033
R Core Development Team (2018). R: A Language and Environment for Statistical Computing. Vienna, Austria: R Foundation for Statistical Computing. Available online at: https://www.R-project.org/
Ryan, M. J., and Brenowitz, E. A. (1985). The role of body size, phylogeny, and ambient noise in the evolution of bird song. Am. Nat. 126, 87–100. doi: 10.1086/284398
Schmidt, A. K. D., Römer, H., and Riede, K. (2013). Spectral niche segregation and community organization in a tropical cricket assemblage. Behav. Ecol. 24, 470–480. doi: 10.1093/beheco/ars187
Sinsch, U., Lumkemann, K., and Rosar, K. (2012). Acoustic niche partitioning in an anuran community inhabiting an Afromontane wetland (Butare. Rwanda). Afr. Zool. 47, 60–73. doi: 10.3377/004.047.0122
Slabbekoorn, H. (2004). “Singing in the wild: the ecology of birdsong,” in Nature’s Music: The Science of Birdsong, eds P. Marler and H. Slabbekoorn (San Diego: Academic Press), 178–205. doi: 10.1016/b978-012473070-0/50009-8
Stiles, G. F., and Skutch, A. F. (1989). A Guide To The Birds Of Costa Rica. New York: Cornell University Press.
Sueur, J. (2002). Cicada acoustic communication: potential sound partitioning in a multispecies community from Mexico (Hemiptera: Cicadomorpha: Cicadidae). Biol. J. Linn. Soc. 75, 379–394. doi: 10.1111/j.1095-8312.2002.tb02079.x
Suthers, R. A., and Zollinger, S. A. (2008). “From brain to song: the vocal organ and vocal tract,” in Neuroscience of Birdsong, eds P. H. Zeigler and P. Marler (Cambridge: Cambridge University Press), 78–98.
Suzuki, R., Taylor, C. E., and Cody, M. L. (2012). Soundspace partitioning to increase communication efficiency in bird communities. Artifi. Life Robot. 17, 30–34. doi: 10.1007/s10015-012-0014-8
Thorpe, W. H. (1963). Antiphonal singing in birds as evidence for avian auditory reaction time. Nature 197, 774–776. doi: 10.1038/197774a0
Tobias, J. A., Planqué, R., Cram, D. L., and Seddon, N. (2014). Species interactions and the structure of complex communication networks. Proc. Natl. Acad. Sci. 111, 1020–1025. doi: 10.1073/pnas.1314337111
Todt, D., and Naguib, M. (2000). Vocal interactions in birds: the use of song as a model in communication. Adv. Stud. Behav. 29, 247–296. doi: 10.1016/s0065-3454(08)60107-2
Villanueva-Rivera, L. J. (2014). Eleutherodactylus frogs show frequency but no temporal partitioning: implications for the acoustic niche hypothesis. PeerJ 2:e496. doi: 10.7717/peerj.496
Wiley, R. H., and Richards, D. G. (1982). “Adaptations for acoustic communication in birds: sound transmission and signal detection,” in Acoustic Communication In Birds, Vol. 1, eds D. E. Kroodsma and E. H. Miller (New York: Academic Press), 132–181.
Wolffgramm, J., and Todt, D. (1982). Pattern and time specificity in vocal responses of blackbirds (Turdus merula). Behaviour 81, 264–286. doi: 10.1163/156853982x00166
Yang, X., Ma, X., and Slabbekoorn, H. (2014). Timing vocal behaviour: experimental evidence for song overlap avoidance in Eurasian wrens. Behav. Process. 103, 84–90. doi: 10.1016/j.beproc.2013.11.011
Keywords: acoustic niche hypothesis, birdsong, spectral and temporal overlap, acoustic signaling, temporal partitioning, acoustic clustering hypothesis
Citation: Hart PJ, Ibanez T, Paxton K, Tredinnick G, Sebastián-González E and Tanimoto-Johnson A (2021) Timing Is Everything: Acoustic Niche Partitioning in Two Tropical Wet Forest Bird Communities. Front. Ecol. Evol. 9:753363. doi: 10.3389/fevo.2021.753363
Received: 04 August 2021; Accepted: 16 September 2021;
Published: 15 October 2021.
Edited by:
Susan Fuller, Queensland University of Technology, AustraliaReviewed by:
Elizabeth Znidersic, Charles Sturt University, AustraliaAnne Clary Axel, Marshall University, United States
Copyright © 2021 Hart, Ibanez, Paxton, Tredinnick, Sebastián-González and Tanimoto-Johnson. This is an open-access article distributed under the terms of the Creative Commons Attribution License (CC BY). The use, distribution or reproduction in other forums is permitted, provided the original author(s) and the copyright owner(s) are credited and that the original publication in this journal is cited, in accordance with accepted academic practice. No use, distribution or reproduction is permitted which does not comply with these terms.
*Correspondence: Patrick J. Hart, pjhart@hawaii.edu
†Present address: Thomas Ibanez, AMAP, Université de Montpellier, CIRAD, CNRS, INRAE, IRD, Montpellier, France; Kristina Paxton, Hawai‘i Cooperative Studies Unit, University of Hawai‘i at Hilo, Hilo, HI, United States; Esther Sebastián-González, Departamento de Ecología, Universidad de Alicante, Alicante, Spain