Function and Clinical Significance of Circular RNAs in Thyroid Cancer
- Department of Endocrinology, First Affiliated Hospital of Anhui Medical University, Hefei, China
Thyroid cancer (TC) is the leading cause and mortality of endocrine malignancies worldwide. Tumourigenesis involves multiple molecules including circular RNAs (circRNAs). circRNAs with covalently closed single-stranded structures have been identified as a type of regulatory RNA because of their high stability, abundance, and tissue/developmental stage-specific expression. Accumulating evidence has demonstrated that various circRNAs are aberrantly expressed in thyroid tissues, cells, exosomes, and body fluids in patients with TC. CircRNAs have been identified as either oncogenic or tumour suppressor roles in regulating tumourigenesis, tumour metabolism, metastasis, ferroptosis, and chemoradiation resistance in TC. Importantly, circRNAs exert pivotal effects on TC through various mechanisms, including acting as miRNA sponges or decoys, interacting with RNA-binding proteins, and translating functional peptides. Recent studies have suggested that many different circRNAs are associated with certain clinicopathological features, implying that the altered expression of circRNAs may be characteristic of TC. The purpose of this review is to provide an overview of recent advances on the dysregulation, functions, molecular mechanisms and potential clinical applications of circRNAs in TC. This review also aimes to improve our understanding of the functions of circRNAs in the initiation and progression of cancer, and to discuss the future perspectives on strategies targeting circRNAs in TC.
Introduction
Thyroid cancer (TC) is the most common pervasive endocrine malignancy, especially in women (Kim et al., 2020). From 1990 to 2017, the incidence and mortality rates of TC has been increasing (Deng et al., 2020). In addition, the incidence and mortality rates of TC are still rapidly increasing, especially in many developed countries, with up to 586,202 newly diagnosed cases and 43,646 global deaths according to the estimates from Global Cancer Statistics in 2020 (Sung et al., 2021). By implementing early detection and optimal treatments, the survival rate of differentiated thyroid cancers (DTCs) has significantly improved. Patients diagnosed with early stage DTCs can achieve 5-years survival rates of approaching 98% and have a recurrence rate of less than 5–10% (Tuttle, 2018; Wang J. et al., 2020). However, the prognosis of patients with TC at an advanced-stage of the disease and multiple organ metastasis remains poor, with a 5-years survival rate of only 15.3% (Wang et al., 2014). Anaplastic TC (ATC), which accounts for 2% or fewer TC cases, is one of the most aggressive human malignancies and has a dismal prognosis with a median survival rate of less than 1 year (Keutgen et al., 2015; Yoo et al., 2019). To prolong the survival time and improve the quality of life of patients with TC, studies aiming to elucidate the tumourigenesis and molecular mechanisms of TC and to identify novel biomarkers and therapeutic targets for TC recurrence and metastasis are urgently needed.
Circular RNAs (circRNAs) are covalently closed single-stranded RNA molecules that have unique properties and powerful biological functions. In 1976, Sanger et al. first discovered single-stranded circRNA molecules in plant-based viruses (Sanger et al., 1976). Using electron microscopy, circRNAs have been identified in eukaryotes and humans as endogenous RNA (Hsu and Coca-Prados, 1979; Kos et al., 1986). However, circRNAs are mainly misinterpreted as non-functional products of pre-mRNA mis-splicing and only a few circRNAs (e.g. circSRY) are thought to have possible functions (Capel et al., 1993). In 2012, Salzman et al. found that circRNAs were the predominant transcript isoform in hundreds of human genes (Salzman et al., 2012). Subsequently, the identification and functional characteristics of ciRS-7 (also known as CDR1as), serving as the efficient miRNA sponges, formed a large class of post-transcriptional regulators (Hansen et al., 2013; Memczak et al., 2013). With the advancement of high-throughput RNA sequencing (RNA-seq) and bioinformatics algorithms, thousands of circRNAs have been identified to have tissue (Xia et al., 2017)/cell (Salzman et al., 2012)/development stage-specific (Chen B. J. et al., 2019) expression patterns in eukaryotes such as human, mice and zebrafish (Wesselhoeft et al., 2018). Several studies have been performed to explore the expression profiles of circRNAs in different cell types and diseases, and the outcomes have completely changed our view of circRNAs, which were originally thought to be junk by-products in the process of gene transcription (Goodall and Wickramasinghe, 2021). Numerous studies have focused on the potential role of circRNAs as promising disease biomarkers. Thousands of circRNAs have been identified as either oncogenes or tumour suppressors that mediate tumourigenesis, metastasis, and chemoradiation resistance in several cancers (e.g. TC, colorectal cancer, and renal cancer) (Hu Z. et al., 2020; Hanniford et al., 2020; Chen J. et al., 2021; Cen et al., 2021). In this review, we summarise the circRNAs involved in TC and their relevant clinical characteristics. A comprehensive understanding of circRNAs may provide valuable clues and useful information for future clinical applications of TC.
Overviews of circRNAs
CircRNA Biogenesis and Characteristics
Most circRNAs are derived from known protein-coding genes with highly active promoters and consist of a single or multiple exons (Enuka et al., 2016). CircRNAs are primarily generated from primary transcripts through back-splicing (Figure 1A) and lariat-driven circularisation, which occurs in exon-skipping events (Figure 1B, left) (Barrett et al., 2015) or during intron removal from pre-mRNAs (Figure 1B, right) (Zhang et al., 2013). These models of circRNAs biogenesis are differ from the canonical linear splicing mechanism (Figure 1) (Li J. et al., 2020; Chen, 2020). Furthermore, circRNAs are resistant to degradation by exonucleases and are more stable than linear RNAs because of their covalently closed ring structures (Suzuki and Tsukahara, 2014). The most common circRNAs are exonic circRNAs (EcircRNAs), whereas the remaining circRNAs are intronic circRNAs (ciRNAs), exon-intron circRNAs (EIciRNAs), mitochondria-encoded circRNAs (MecciRNAs), and circRNAs of pre-tRNA splicing (TricRNAs) (Figure 1) (Chen, 2020).
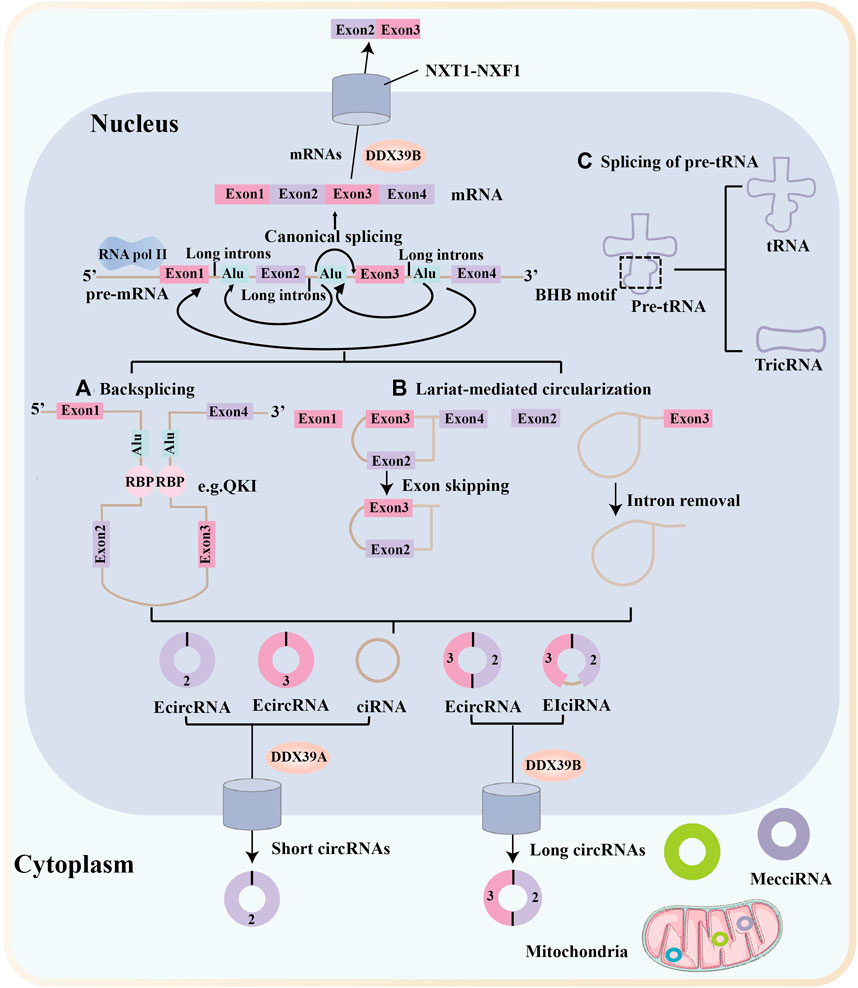
FIGURE 1. Biogenesis and nuclear export of circular RNAs. Messenger RNA (mRNA) synthesis occurs via canonical splicing, in which exons are aligned to generate the mRNA. Most circular RNAs (circRNAs) are transcribed by RNA polymerase II (Pol II) and formed by back-splicing of precursor mRNAs. Many circRNAs, especially those of low abundance, are formed as a result of base pairing between long flanking complementary introns containing inverted repeat elements, such as Alu repeats. (A) CircRNA biogenesis is fine-tuned by trans-acting RNA binding proteins (RBPs). (B) Another circRNA biogenesis model is the lariat-driven circularization, which occurs in exon-skipping events (left) or during intron removal from pre-mRNAs (right). (C) TricRNAs are another type of circRNA that are generated via the splicing of pre-tRNA. MecciRNAs are mitochondria-encoded circRNAs that are distributed in the mitochondria and the cytoplasm. Export of circRNAs from the nucleus require various proteins and occur in a length-dependent manner. DDX39B regulate the nuclear export of long circRNAs (>1,300 nucleotides), whereas DDX39A regulate the nuclear export of short circRNAs (< 400 nucleotides). NTF2-related export protein 1 (NXT1)-nuclear RNA export factor 1 (NXF1) heterodimeric export receptor recruit some complexes and release into the cytoplasm.
Recent research into circRNA biogenesis has shown that back-splicing is catalysed by the canonical spliceosomal machinery and modulated by both intronic complementary sequences (ICSs) and RNA binding proteins (RBPs) (Li et al., 2018c). Pairing between ICSs on different introns is considered to bring the distal splicing sites closer, thereby enhancing back-splicing (Zhang et al., 2016). RBPs usually modulate back-splicing by directly connecting distal splice sites and binding to ICSs sites (Li et al., 2017; Okholm et al., 2020). For example, protein quaking (QKI) enhances circRNA formation by binding to its recognition motif in introns flanking circRNA-forming exons (Conn et al., 2015).
Similar to many linear mRNAs, circRNAs containing introns are frequently sequestered in the nucleus, but most circRNAs accumulate in the cytoplasm (Patop et al., 2019; Goodall and Wickramasinghe, 2021). A study by Huang et al. showed that a length-dependent evolutionarily conserved pathway mediated by DDX39B or DDX39A controls the nuclear export of circRNAs (Huang et al., 2018). Another study showed that the nuclear export of circNSUN2 was mediated by the m6A-binding protein YTHDC1, providing the first evidence that m6A controls circRNA translocation (Chen R.-X. et al., 2019).
CircRNA Degradation and Exosomes Release
CircRNAs are resistant to degradation owing to their stable structure and the mechanisms underlying their degradation have only recently begun to be elucidated (Figure 2) (Li J. et al., 2020). Some circRNAs are degraded upon miRNA binding and argonaute-2 (AGO2) mediated cleavage (Figure 2A) (Hansen et al., 2011), whereas others are degraded by endoribonucleolytic cleavage by the endoribonuclease complex RNase P/MRP following modification with N6-methyladenosine (m6A) (Figure 2B). This degradation is mediated by the m6A reader protein YTHDF2 and adaptor protein HRSP12 in the cytoplasm (Park et al., 2019). Another decay mechanism involves ribonuclease L (RNase L) and double-stranded RNA-activated protein kinase R (PKR). Upon poly (I:C) stimulation or viral infection, RNase L is induced to degrade circRNAs, thereby releasing and activating PKR, which play a role in the early response of innate immunity (Figure 2D) (Liu C.-X. et al., 2019). Moreover, some RBPs are associated with the secondary structure of circRNAs. For example, upstream frameshift 1 (UPF1) and its associated endonuclease G3BP1 bind and unwind circRNAs, and the helicase activity of UPF1 leads to circRNA degradation (Figure 2C) (Fischer et al., 2020). Recently, it was reported that RNase H1 is responsible for nuclear circRNA degradation (Figure 2E) (Li et al., 2021c). This mechanism limits ciRNA accumulation by recruiting RNase H1 and resolves R-loops for transcriptional elongation at some GC-rich ciRNA-producing loci; one ciRNA, ciankrd52 with a locally open RNA structure, shows a stronger ability of R-loop formation and degradation by RNase H1 cleavage (Li et al., 2021c).
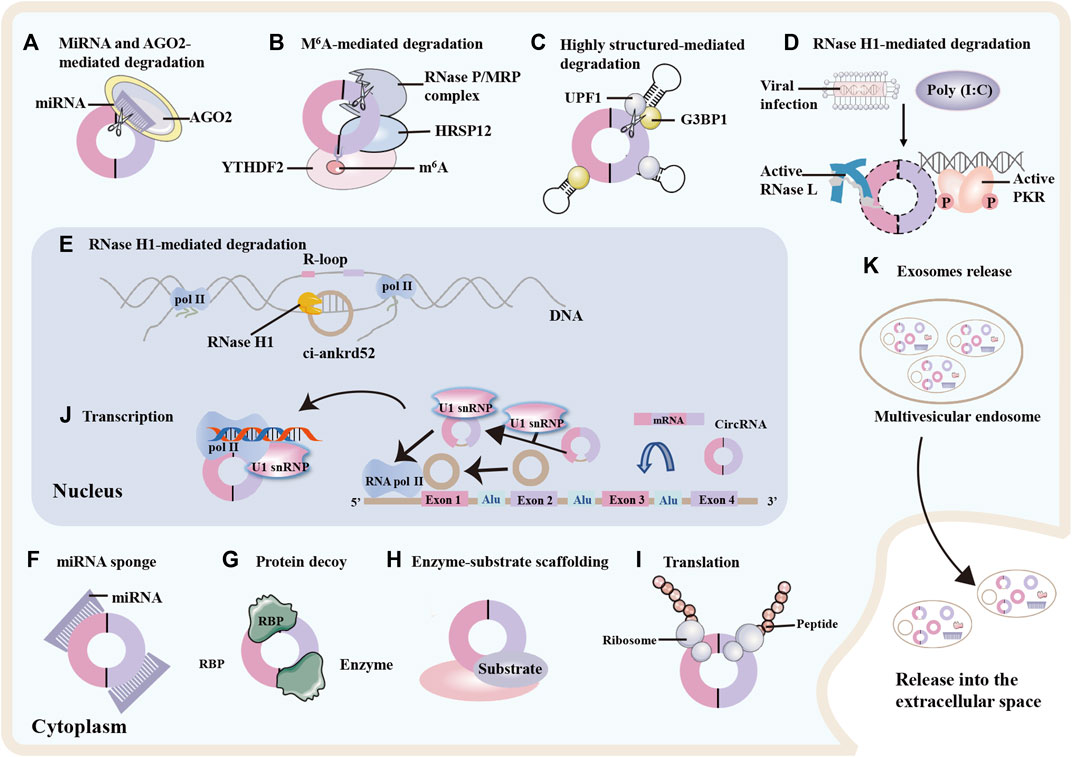
FIGURE 2. circRNAs degradation, functions and exosomes release. Degradation of circRNAs: (A) Specific microRNA (miRNA) direct binding to circular RNA (circRNA) facilitates cleavage of the circRNA by argonaute 2 (AGO2). (B) N6-methyladenosine (m6A)-modified circRNAs can be cleaved by the YTHDF2-HRSP12-RNase P/MRP complex. (C) The RNA-binding proteins UPF1 and its associated protein G3BP1 bind and regulate circRNA with highly-structured 3′UTRs, promoting their cleavage by the helicase activity of UPF1. (D) Upon viral infection, RNase L is activated and degrades the circRNAs, thereby releasing and activating protein kinase R (PKR) and decreasing global circRNA levels. (E) In the nucleus, RNase H1 degrades ciankrd52 dependent upon R-loop, thereby promoting RNA polymeraseII (Pol II) transcriptional elongation at ciRNA-producing loci. CircRNAs functions. (F) circRNAs can function as miRNA sponge by binding miRNA and inhibiting the expression of miRNA-mediated target messenger RNA (mRNA). (G) circRNAs can interact with RNA binding proteins (RBPs) and influence protein expression and function. (H) circRNAs can act as protein scaffolds for enzyme-substrate to modulate protein-protein interactions. (I) circRNAs with ribosome entry sites can be translated into proteins. (J) In the nucleus, circRNAs can interact with small nuclear ribonuleoprotein U1 (U1 snRNP) or promote Pol II elongation machinery to enhance RNA Pol II, then promoting gene expression. Exosomes release. (K) circRNAs can be enriched in exosomes and released into the extracellular environment upon exocytic fusion of multivesicular endosomes with the cell surface.
Some circRNAs are generally wrapped in multivesicular endosomes (40–160 nm) and secreted from various cells upon fusion of multivesicular bodies with the cell membrane (Figure 2K) (Kalluri and LeBleu, 2020; Seimiya et al., 2020). When using a stringent spliced reads per billion mapping cutoff, 1,428 of exosomal circRNAs and only 319 of cellular circRNAs were confirmed. This data suggest that the number of circRNAs in exosomes is on average higher than in the cancer cells from which they were released (Li Y. et al., 2015). Accumulating evidence indicates that exosomes play an important role in cancer progression, metastasis, and drug resistance (Xie et al., 2022; Yang et al., 2022). To date, exosomes have been detected in the plasma of patients with TC and carry biological effectors that contribute to the progression (Wu et al., 2019). Consequently, plasma exosomal circRNAs may be promising non-invasive biomarkers of TC.
CircRNAs Functions
Based on the localisation of circRNAs, van Zonneveld et al. summarised and classified them into two categories: cytoplasmic circRNAs and nuclear-enriched circRNAs (van Zonneveld et al., 2021). The mechanisms of cytoplasmic circRNA function include (I) acting as miRNA and protein sponge (II) functioning as protein scaffolds, and (III) acting as a template for protein translation. Some circRNAs act as regulators of gene expression and have been identified in the nucleus (van Zonneveld et al., 2021).
Various cytoplasmic circRNAs have been reported to function as decoys for miRNAs and proteins, scaffolds for proteins, and templates for protein translation (Greene et al., 2017; Seimiya et al., 2020). To date, the most essential function of circRNAs is a miRNA sponge (Figure 2F). In the cytoplasm, some circRNAs serve as competing endogenous RNAs (ceRNAs), defined as miRNA sponges that block the regulation of miRNA on their target sites and affect gene expression and transcription regulation. For example, circLDLR behaves as a ceRNA sponge for miR-195-5p, resulting in a decreased miR-195-5p function and upregulated miR-195-5p target genes in papillary thyroid cancer (PTC) (Gui et al., 2020). Additionally, circRNAs often engage with numerous RBPs by acting as protein decoys (Figure 2G) and scaffolds (Figure 2H) to regulate protein functions and enhance the reaction kinetics of enzyme-substrate interactions (Du et al., 2016; Zang et al., 2020). For instance, circRNA_102,171 was found to promote the growth and invasion of PTC cells by binding to the β-catenin interacting protein 1 (CTNNBIP1) (Bi et al., 2018). In addition to protein sponging, circfoxo3 scaffolded p21 and cell cycle protein dependent kinase 2 to inhibit cell cycle progression (Du et al., 2016).
Recently, circRNAs (e.g., circZNF609) containing internal ribosome entry sites (IRESs) were found to be translated into proteins in eukaryotes (Chen et al., 2016; Legnini et al., 2017). In addition, some circRNAs serve as sources of pseudogene generation, modulating gene expression in the nucleus (Li Z. et al., 2015). For example, some nuclear EIciRNA (e.g., circEIF3J and circPAIP2) can enhance Pol II expression, thereby regulating gene expression at transcriptional and post-transcriptional levels (Figure 2J) (Li Z. et al., 2015).
To date, Numerous studies have shown the broad expression of endogenous circRNAs in all human tissues and circRNAs have been increasingly implicated in the regulation of cell proliferation, tumourigenesis, autophagy, neuronal functions and immune systems through various molecular mechanisms (Chen, 2020). However, biological functions have only been investigated for a minor fraction of the circRNAs identified to date, most of which still require further studies.
CircRNAs Expression Profiles in TC
RNA-seq, circRNA-specific microarrays and bioinformatics analyses are the most commonly used methods for genome-wide profiling of circRNAs, and thousands of circRNAs have been identified in tissues, cells, exosomes, and blood of patients with TC (Table 1) (Peng et al., 2017; Hou et al., 2018; Lan et al., 2018b; Ren et al., 2018; Yang et al., 2019; Chu et al., 2020b; Guo et al., 2020a; Liu et al., 2020b; Liu Q et al., 2020; Long et al., 2020; Sun J. W. et al., 2020; Yang W. et al., 2020; Yang Y. et al., 2020; Yu et al., 2020; Chu et al., 2021; Guo et al., 2021; Li et al., 2021b; Lv et al., 2021; Qiu et al., 2021; Zhang et al., 2022). Hundreds of differentially expressed circRNAs (DECs) were identified between the tumour and non-tumour groups. For example, Peng et al. identified 453 circRNAs that were expressed in 6 matched PTC samples compared to control; 217 circRNAs were significantly upregulated, and 236 circRNAs were downregulated (Peng et al., 2017). Among four studies, the microarray dataset GSE93522 was the most commonly used database for secondary bioinformatic analyses intended to identify novel circRNAs for further research (Peng et al., 2017; Liu Q. et al., 2020; Li et al., 2021b; Qiu et al., 2021). Among these studies, circHACE1 was significantly downregulated (Li et al., 2021b) and hsa_circ_0004458 was upregulated in PTC tissues (Liu Q. et al., 2020). circ_0004053 and circ_0028198 was upregulated in PTC compared to that in normal samples (Qiu et al., 2021). Furthermore, researchers have focused on the differential expression and potential role of circRNAs in TC cell lines (Hou et al., 2018; Jiang et al., 2018; Long et al., 2020). Yu et al. detected 392 DECs between primary and lymph node metastasis (LNM) tumours, and of these DECs, circRNA-UMAD1 was selected as a sponge for miR-873 and was correlated with Gal3 levels in peripheral circulation (Yu et al., 2020). Exosomes have been reported to participate in intercellular communication by transmitting their cargo, including miRNAs, lncRNAs, proteins and even circRNAs to recipient cells, thereby regulating tumour progression (Zhou H. et al., 2021; Jafari et al., 2021). Yang et al. identified three differentially regulated circRNAs included hsa_circ_007,293, hsa_circ_031752, and hsa_circ_02013 in serum exosomes from patients with PTC compared with controls (Yang et al., 2019). These circRNAs (e.g., circFNDC3B) might be potential liquid biopsy indicators for the diagnosis of TC and may play regulatory roles in the progression of TC (Wu et al., 2020b).
Biological Functions of circRNAs in TC
Oncogenic Activity of circRNAs
A growing body of evidence has confirmed that upregulated circRNAs function as oncogenes involved in the occurrence and progression TC by regulating malignant cell phenotypes, including cell colony formation, proliferation, migration, invasion and epithelial-mesenchymal transition (EMT) (Supplementary Material S1, Figure 3).
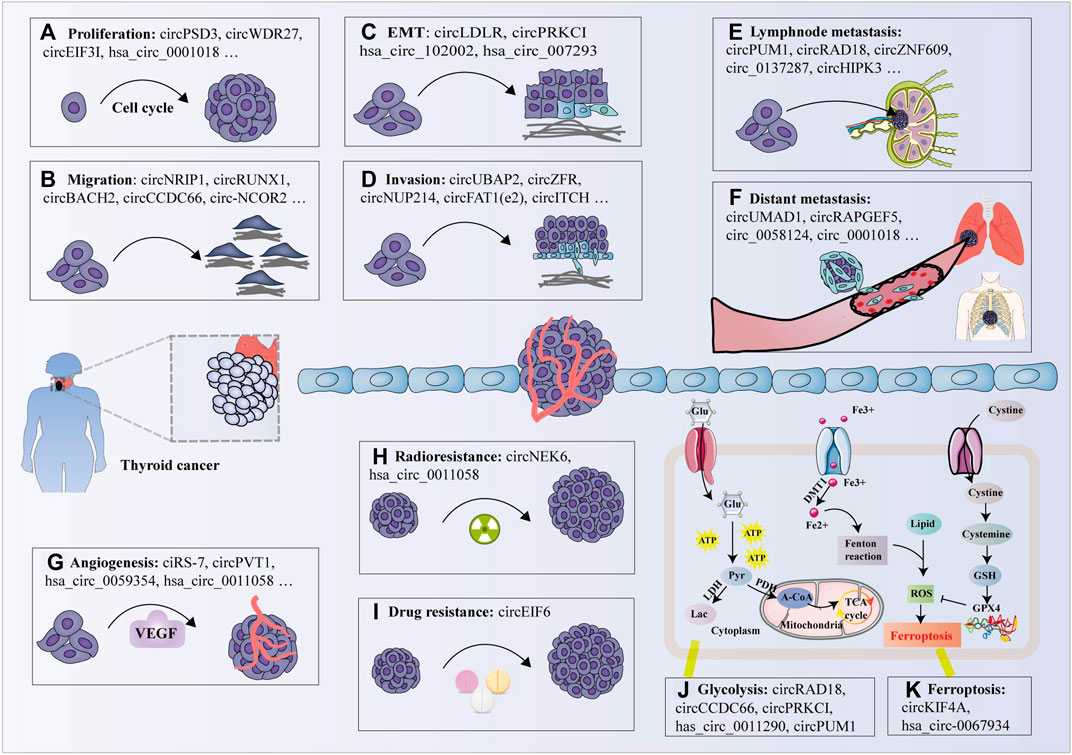
FIGURE 3. Biological functions of circRNAs in thyroid cancer. (A) circRNAs promote cell proliferation by promoting (e.g., circPSD3) or inhibiting (e.g., circITCH); (B) circRNAs promote cell migration by facilitating (e.g., circNRIP1) or inhibiting (e.g., hsa_circ_0007694); (C) circRNAs modulate the epithelial-mesenchymal transition (EMT) process by promoting (e.g., circLDLR); (D) Some circRNAs promote cell invasion (e.g., circZFR), while other circRNAs inhibit cell invasion (e.g., circNEURL4); (E) Several circRNAs are correlated with lymphnode metastasis (e.g., circPUM1); (F) A few circRNAs are associated with distant metastasis (e.g., circUMAD1); (G) Some circRNAs have been shown to promote tumor angiogenesis (e.g., ciRS-7) by modulating vascular endothelial growth factor A (VEGFA) expression; (H) Several circRNAs facilitate radioresistance in TC cells (e.g., circNEK6); (I) Individual circRNA promotes the drug-resistance of TC cells (e.g., circEIF6); (J) Certain circRNAs modulate glycolysis (e.g., circRAD18); and (K) ferroptosis (e.g., circKIF4A) in thyroid cancer cells. Glu: glucose, ATP: adenosine triphosphate, Pyr: pyruvate, Lac: lactate, LDH: lactate dehydrogenase, PDH: pyruvate dehydrogenase, A-CoA: acetyl-CoA, TCA cycle: tricarboxylic acid cycle, GSH: glutathione, ROS: reactive oxygen species, GPX4: glutathione peroxidase 4.
To date, numerous circRNAs have been proposed to bind to various miRNAs and inhibit their mRNA activity via a function known as miRNA spongs or decoys (Supplementary Materials S1,2). Studies suggested that identical circRNAs contain multiple miRNA-binding sites that can perform various functions by sponging different miRNAs and inhibiting their mRNA activity (Qi et al., 2021b), as exemplified by circPSD3, which contains target sites for miR-7-5p, miR-885-5p and miR-637 (Jin et al., 2018; Li et al., 2021e; Zhu et al., 2021). Studies have also revealed that different circRNAs contain the same type and miRNA binding sites that can specifically bind to miRNAs, thereby reducing miRNA activity and upregulating the expression of miRNA-related target genes (Shu et al., 2020; Luo et al., 2021), such as hsa_circ_0058124 and circUBAP2, which are revealed as miR-370-3p sponges and promote proliferation and invasion of TC cells (Liu L. et al., 2020; Xiong et al., 2021b). In addition, specific circRNAs protect homologous mRNAs from miRNA-mediated degradation by inhibiting miRNA activity (Zeng et al., 2021). For example, Zeng et al. revealed that circPVT1 serves as a ceRNA to sequester miR-195 and promote the PVT1-mediated malignant progression in PTC (Zeng et al., 2021). Accumulating evidence has identified individual circRNAs containing multiple RBP motifs, suggesting circRNAs may sponging protein and modulate RBP-dependent functions (Bronisz et al., 2020; Tsitsipatis et al., 2021). For instance, circ_102171 has been shown to accelerate the malignant behaviour of PTC cells by interacting with CTNNBIP1 and regulating the Wnt/β-catenin signalling way. Silencing of circ_102171 suppressed PTC cell proliferation, migration and invasion, while promoting apoptosis in vitro and inhibiting PTC growth in vivo (Bi et al., 2018). In addition, certain circRNAs promote angiogenesis in TC (Li S. et al., 2020; Zeng et al., 2021). A tube formation assay showed that circ_0011058 knockdown notably decreased fibroblast growth factor 2 and vascular endothelial growth factor A, which are important activators of angiogenesis, thereby impeding the proliferation and angiogenesis of PTC cells (Zhang Z. et al., 2021).
Anti-Tumour Activity of circRNAs
Generally, six downregulated circRNAs (e.g., circHACE1, circITCH, circNEURL4, hsa_circ_100395, hsa_circ_0007694, circSH2B3) function as tumour suppressors in TC, inducing cell cycle arrest and apoptosis while hampering cell proliferation, migration, and invasion (Peng et al., 2017; Wang M. et al., 2018; Long et al., 2020; Ding W. et al., 2021; Li et al., 2021b; Sa et al., 2021) (Supplementary Materials S1,S2, Figure 3). In addition, recent studies have identified that several upregulated circRNAs are involved in cell signal transduction, which is a process of transferring molecular signals from the extracellular space into the cell through the cell membrane, thereby inducing the tumourigenesis of PTC (Zhou et al., 2018; Yao et al., 2019). For example, Wang et al. observed that circ-ITCH overexpression significantly inhibited the proliferation and invasion of PTC cells by upregulating the expression of CBL and promoting apoptosis in vitro, which led to suppression of the Wnt/β-catenin pathway and the tumour-suppressive role of circ-ITCH (Wang M. et al., 2018).
Modulating Radioresistance
Radioactive iodine (RAI) is used after thyroidectomy to ablate the residual normal thyroid remnant, as adjuvant therapy, and to treat TC (Luster et al., 2014). Problematically, it has been reported that approximately 30% of advanced DTC will eventually lose the ability to concentrate radioiodine and dedifferentiate due to decreased expression of Na/I symporter (NIS) (Trouttet-Masson et al., 2004; Woodrum and Gauger, 2005). Reestablishing the ability to concentrate iodine and redifferentiation becomes the principal problem faced by the radioactive iodine therapy for poorly DTC. Accumulating evidence suggests that some circRNAs play increasingly important roles in the regulation of radioaction responses (Supplementary Material S1, Figure 3H) (Gu et al., 2021; Wu et al., 2021). For example, Chen et al. observed that circ_NEK6 expression was elevated in 131I-resistant DTC tissues and cell lines, and knockdown of circ_NEK6 repressed 131I resistance in DTC and suppressed cell proliferation, migration, and invasion abilities while inducing cell apoptosis and DNA damage (Chen F. et al., 2021). Furthermore, Sa et al. first identified that downregulation of circSH2B3 increased 125I uptake and NIS expression levels in PTC cells treated with aryl hydrocarbon receptor (AhR) antagonists, which has been implicated in the dedifferentiation of radioiodine-refractory PTC (Sa et al., 2021). These results support the evidence that circRNAs directly or indirectly mediate radioresistance by forming a ceRNA network and may function as therapeutic targets to improve the efficacy of refractory/relapsed patients.
Regulating Chemoresistance
Drug treatment together with surgical operation, radiotherapy and biotherapy constitute the main approaches to cancer treatment (Jaaks et al., 2022). With the clinical application of anti-tumour molecular targeting drugs, the survival rate of patients with tumours have been significantly extended (Stone et al., 2017). However, chemoresistance remains an intractable problem that hinders better patient prognosis (Herzog et al., 2021; Lampropoulou et al., 2022). Accumulating evidence suggests that ncRNAs, including miRNAs, lncRNAs, and circRNAs, may drive drug resistance in various cancers, including TC (Supplementary Material S1, Figure 3I) (Liu F. et al., 2018; Gao et al., 2020; Zhang H. et al., 2021; Lampropoulou et al., 2022). Liu et al. showed that hsa_circ_0060060 (circEIF6) overexpression was negatively correlated with miR-144-3p and enhanced cisplatin resistance by autophagy activation in TPC1 and BHT101 cells, suggesting that circEIF6 plays a crucial role in cisplatin resistance (Liu F. et al., 2018). Currently, studies of circRNAs in chemoresistance are rare, and further investigations are needed to explore the detailed mechanisms and potential clinical applications.
Regulation of TC Metabolism
Deregulated metabolism, which is widespread in tumor progression, provides an essential source for proliferation and growth of cancer cells. Glycolysis, fatty acid oxidation, and amino acid metabolism are responsible for metabolic reprogramming of cancer cells (Stine et al., 2022). Under adequate oxygen condition, cancer cells increase glucose uptake and ATP and lactic acid accumulation through glycolysis. This phenomenon is termed as aerobic glycolysis or the Warburg effect (Warburg et al., 1927). Some circRNAs promote the Warburg effect and regulate the malignant behaviour of many tumours by sponging miRNAs (Chen X. et al., 2019; Cao et al., 2020). Targeting the intrinsic metabolism of cancer cells has proven to be a promising therapeutic strategy for TC (Supplementary Material S1, Figure 3J) (Liu Y. et al., 2021; Zhang Q. et al., 2021). Pyruvate dehydrogenase kinase (PDK) is a critical modulator of key glycolysis enzymes and is associated with EMT, poor prognosis and therapy resistance (Atas et al., 2020). A recent study confirmed that silencing circRAD18 remarkably inhibited cell glucose uptake, lactate production and the expression level of PDK1 protein in PTC cells, indicating the regulatory effect of circRAD18 on glucose metabolism reprogramming in PTC (Chen et al., 2021e). Consistent with these findings, knockdown of circCCDC66 suppressed the glycolytic metabolism of TC by targeting the miR-211-5p/PDK4 axis (Ren et al., 2021). Moreover, alterations in fatty acid metabolism can influence energy storage, affect drug resistance, modulate cell proliferation and survival, and stimulate the extracellular environment (Röhrig and Schulze, 2016). Wen et al. identified four recurrence-related genes (PDZK1IP1, TMC3, LRP2 and KCNJ13) and established a four-gene signature recurrence risk model, indicating that lipid metabolism-related gene profiling represents a potential marker for prognosis and treatment decisions for PTC patients (Wen S. et al., 2021). Nevertheless, the mechanism of circRNAs in lipid metabolism of TC remains largely unknown and is expected to become a novel field in the study of circRNAs in TC.
Function of circRNAs in Ferroptosis and Other Mechanisms
Ferroptosis is an iron- and reactive oxygen species (ROS)-dependent form of cell death, characterised mainly by cytological changes (Huang et al., 2021). Accumulating evidence suggests that circRNAs may function as essential regulators of ferroptosis in cancers, including TC (Supplementary Material S1, Figure 3K) (Wang H.-H. et al., 2021; Chen et al., 2021d; Yang et al., 2021). For example, Wang et al. observed that silencing circ_0067934 increased the levels of ferroptosis-related markers, including Fe2+, iron, and ROS, in TC cells, suggesting that circ_0067934 may serve as a potential therapeutic target by regulating ferroptosis for the treatment of TC (Supplementary Material S1, Figure 3K) (Wang H.-H. et al., 2021). In addition, individual circRNAs may modulate the expression of apoptosis-related proteins (e.g., Bax and caspase-3) (Xia et al., 2020), metastasis-associated protein (MTA2, MTA) (Yang Y. et al., 2020; Luan et al., 2020), and epithelial mesenchymal phenotype biomarkers (MMP2, MMP9, Twist1, E-cadherin, N-cadherin, vimentin, and Slug) (Han J.-y. et al., 2020; Gui et al., 2020; Xia et al., 2020; Zhang W. et al., 2021; Wang W. et al., 2021) to mediate cell apoptosis, metastasis, and EMT. In addition, a few circRNAs may indirectly activate or inactivate several vital signaling pathways by suppressing miRNAs, such as the NOTCH3/GATAD2A (Yao et al., 2019), JAK/STAT/AMPK (Cui and Xue, 2020), PI3K/AKT/mTOR (Long et al., 2020), and Wnt/β-catenin signalling pathways (Bi et al., 2018; Chen et al., 2018; Long et al., 2020; Zeng et al., 2021) For instance, Cui et al. observed that hsa_circ_100,721 (circDOCK1) serves as a ceRNA for miR-124, leading to dampening signal transduction of the JAK/STAT/AMPK pathway (Cui and Xue, 2020). Dong et al. revealed that circ_0067934 acts as a molecular sponge for miR-1301-3p to induce malignant effects in PTC cells, resulting in the activation of PI3K/Akt and MAPK pathways (Dong et al., 2022). However, the specific mechanisms underlying these circRNA functions remain unknown and require further study.
Potential Application of circRNAs in TC
At around the time when circRNAs were first discovered, Sanger et al. described circRNAs as viroids with pathogenic activity towards certain higher plants (Sanger et al., 1976). However, with in-depth studies of circRNAs, increasing evidence has emphasised that circRNAs are essential for gene expression. CircRNAs are highly abundant and widely distributed in nearly all types of human tissues, cells, and bodily fluids, such as blood (Chen C. et al., 2022), bile (Xu et al., 2021), saliva (Jafari Ghods, 2018), breast milk (Zhou Y. et al., 2021), urine (He et al., 2021), ascites (Du et al., 2022), pleural effusion (Wen et al., 2018), synovial fluid (Wu et al., 2022), cerebrospinal fluid (Wang Z. et al., 2022), and bronchoalveolar lavage fluid (Liu Q.-P. et al., 2021), and are even enriched in exosomes (Fan et al., 2022).
CircRNAs account for approximately 1% of poly(A) RNA in human cells (Jeck and Sharpless, 2014), and over 25,000 distinct circRNAs have been identified in human fibroblasts (Jeck et al., 2013). CircRNAs are prone to detection because of their higher expression in peripheral whole blood compared to linear ncRNAs (Memczak et al., 2015). In addition, circRNAs are resistant to RNase R digestion and can be easily detected using quantitative reverse transcription-polymerase chain reaction (qRT-PCR) assays (Chen L. et al., 2022). Finally, their expression levels are extremely diverse and variable based on the cell type and development stage of the tissues (Chen, 2020). With the advantages detailed above, numerous circRNAs can be characterised as non-invasive and repeatable biomarkers. Here, we used a few typical examples to discuss the clinical implications of specific circRNAs in TC.
CircRNAs as Promising Diagnostic and Prognostic Biomarkers for TC
Compared with normal controls, circRNAs present significantly differential expression profiles in TC tissues and blood from patients with TC; thus, they are regarded as promising and ideal candidates for the diagnosis of TC owing to their abnormal expression and high specificity (Table 2; Figure 4) (Jin et al., 2018; Lan et al. 2018a; Ren et al. 2018; Wang et al. 2018b; Wei et al. 2018; Cai et al. 2019; Yao et al. 2019; Wang et al. 2019a; Fan et al. 2020; Han et al. 2020a; Hu et al. 2020b; Liu et al. 2020b; Shi et al. 2020; Sun et al. 2020b; Wang et al. 2020b; Wu et al. 2020a; Xue et al. 2020; Ye et al., 2020; Yu et al., 2020; Zhang et al. 2020b; Chu et al., 2021; Ding et al. 2021a; Ding et al. 2021b; Du et al. 2021; Guo et al. 2021; Li et al. 2021a; Li et al. 2021b; Li et al. 2021d; Lin et al. 2021; Liu et al. 2021b; Luo et al., 2021; Ma and Kan, 2021;Qi et al., 2021b; Xiong et al., 2021b; Zeng et al., 2021; Zhang et al. 2021c; Zhang et al. 2021d; Zheng et al. 2021a; Zhu et al., 2021; Dong et al., 2022; Li et al. 2022; Nie et al. 2022). For example, Zhang et al. documented significant upregulation of circRNA_103,598 expression in PTC tissues and cell lines, with an area under the receiver operating characteristic (ROC) curve (AUC) as high as 0.9456 (Zhang S. et al., 2020). Sun et al. demonstrated that two circRNAs (hsa_circ_0124055 combined with hsa_circ_0101622) provided a more powerful diagnostic value (AUC = 0.911, 95% CI: 0.859–0.962, p < 0.001) than the use of hsa_circ_0124055 (AUC = 0.836) or hsa_circ_0101622 (AUC = 0.805) alone (Sun JW. et al., 2020).
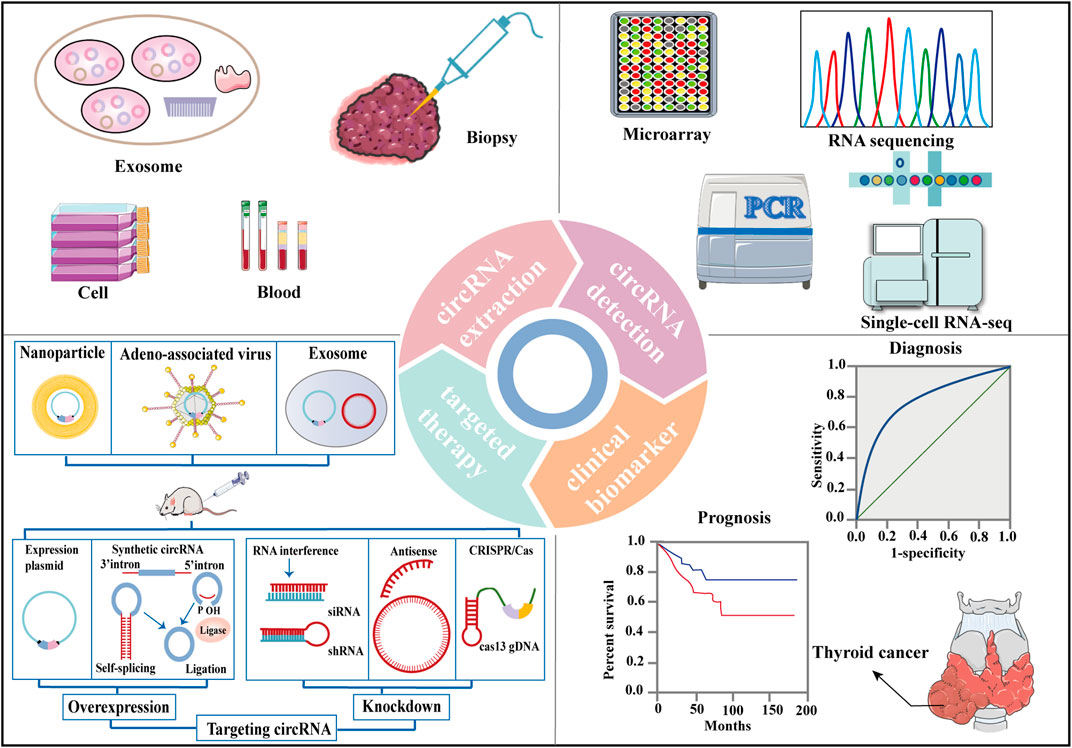
FIGURE 4. Clinical applications of circRNAs in thyroid cancer. CircRNAs are extracted from cell, blood, biopsy and exosome from TC patients. RNA sequencing, microarray, single-cell RNA-seq, quantitative reverse transcriptase-polymerase chain reaction (qRT-PCR) assays are methods that can be used to detect circRNA levels. CircRNAs are significantly associated with many clinicopathologic characteristics of TC and with TC patient survival parameters, rendering them potential diagnostic and prognostic biomarkers for TC. Overexpression or knockdown of target circRNAs resulting from delivery may serve as potential therapeutic approaches for TC.
CircRNAs have been reported to be significantly associated with many clinicopathological characteristics in TC, including tumour size, histological grade, lymph node metastasis (LNM), distant metastasis, multifocality, extrathyroidal extension, invasion and recurrence (Table 2). For example, Ye et al. observed that circFOXM1 is significantly upregulated in PTC tissues and in TPC-1 and BCPAP cells and that circFOXM1 levels are associated with tumor size (p = 0.001), TNM stage (p = 0.002), LNM (p = 0.002), and nodular goiter (p = 0.009) (Ye et al., 2020). In contrast, circ-ITCH is downregulated in PTC tissues and cell lines, and its expression levels are significantly associated with LNM (p = 0.020), clinical stage (p = 0.022) (Wang M. et al., 2018). Similarly, hsa_circ_IPCEF1 is significantly decreased in both PTC tissues and blood, and its levels were positively correlated with LNM (p < 0.001) (Guo et al., 2021). Most studies have reported that there is no relationship between circRNA levels and gender in TC. It should be noted that female have higher incidence and favorable DTC outcomes than male (Zhang D. et al., 2018).
Finally, defining a precise prognosis for TC patients is essential for physicians to formulate the best treatment decisions. To further analyse the prognostic value of circRNAs in TC, we collected information from studies reporting survival information and evaluated the associations between circRNA expression levels and overall survival (OS), disease-free survival (DFS), and progression-free survival (PFS) (Table 2) (Jin et al., 2018; Lan et al. 2018a; Ren et al. 2018; Wang et al. 2018b; Wei et al. 2018; Cai et al. 2019; Yao et al. 2019; Wang et al. 2019a; Fan et al. 2020; Han et al. 2020a; Hu et al. 2020b; Liu et al. 2020b; Shi et al. 2020; Sun et al. 2020b; Wang et al. 2020b; Wu et al. 2020a; Xue et al. 2020; Ye et al., 2020; Yu et al., 2020; Zhang et al. 2020b; Chu et al., 2021; Ding et al. 2021a; Ding et al. 2021b; Du et al. 2021; Guo et al. 2021; Li et al. 2021a; Li et al. 2021b; Li et al. 2021d; Lin et al. 2021; Liu et al. 2021b; Luo et al., 2021; Ma and Kan, 2021;Qi et al., 2021b; Xiong et al., 2021b; Zeng et al., 2021; Zhang et al. 2021c; Zhang et al. 2021d; Zheng et al. 2021a; Zhu et al., 2021; Dong et al., 2022; Li et al. 2022; Nie et al. 2022). For example, Wang et al. observed that circ_0067934 was highly expressed in TC tissues, and Cox proportional hazards regression model analysis indicated that circ_0067934 expression level was independently associated with OS (RR = 4.385, 95%CI = 1.087–17.544, p = 0.038) (Wang H. et al., 2019). Ding et al. revealed that higher circ_0015278 expression was independently correlated with improved DFS (p = 0.026, HR = 0.529) and found that higher pathological tumour-node-metastasis stage was an independent factor of shorter DFS (p = 0.017, HR = 1.766), and tumour size (>4 cm vs≤ 4 cm) as independent factors of unfavourable OS in patients with PTC (p = 0.012, HR = 4.835) (Ding H. et al., 2021). Similarly, a study by Liu et al. identified that higher expression of hsa_circ_0102272 resulted in worse OS and PFS in patients (Liu et al., 2020b).
CircRNAs as Potential Targets for TC
Several oncogenic and antioncogenic circRNAs have been discovered to regulate the initiation and development of TC (Supplementary Material S1). Overexpression or knockdown of related circRNAs might be an effective intervention strategy for TC progression. RNA interference (Wang L. et al., 2018; Cooper et al., 2018), CPISPR/Cas9 editing (Piwecka et al., 2017), plasmid transfection (Tatomer et al., 2017), and lentivitral vector infection (Wang M. et al., 2018; Ding W. et al., 2021; Li et al., 2021b; Sa et al., 2021) are methods that can be used to regulate circRNA levels (Figure 4). Small interfering RNAs (siRNAs) or short hairpin RNAs (shRNAs) that were designed to target the backspliced junction region of oncogenic circRNAs may suppress tumour growth and metastasis in patient-derived xenograft (PDX) mouse models (Zhang Q. et al., 2021; Chu et al., 2021; Zhang W. et al., 2021; Chen et al., 2021d; Wang W. et al., 2021). The synthesis and circRNA sequences were cloned into specific plasmid vectors for the production of lentiviral particles, which stably transfected TC cell lines and expressed the corresponding and desired circRNAs (Figure 4) (Wang M. et al., 2018; Ding W. et al., 2021; Li et al., 2021b; Sa et al., 2021). For example, Li et al. found that the circHACE1 sequence was cloned into the pLO5-ciR vector (Geenseed, Guangzhou, China) for the production of lentiviruses to stably transfect DTC cell lines and then acted as a tumour repressor (Li et al., 2021b). Exogenous circRNAs might be from the transfection of purified in vitro generated circRNAs or delivered by specific vectors containing DNA cassettes, designed for circRNA expression (Li J. et al., 2020). So far, exogenous circRNAs have been successfully loaded into nanoparticles for targeted therapy due to the specific advantages of nanoparticles, such as reduced toxicity and precise targeting (Figure 4) (Aikins et al., 2020). Additionally, drugs or viruses can mediate anti-tumour effects through individual circRNA or circRNA-associated axes (Hou et al., 2018; Zhang S. et al., 2020). Some circRNAs mentioned above, such as circEIF6 (Liu F. et al., 2018), circ_NEK6 (Chen F. et al., 2021), circ_0011058 (Zhang Z. et al., 2021), are related to chemoradiation resistance in TC. Therefore, targeting circRNAs may be important for treating tumour resistance clinically and provide a new approach for TC treatment.
Approaches for circRNA Studies and Future Perspectives
To better study the biological functions and applications of circRNAs, numerous circRNA-associated public databases (e.g., CircBase and Circ2Traits) have been developed to facilitate circRNA analyses (Ghosal et al., 2013; Glažar et al., 2014; Chen L. et al., 2021). Other databases and their common uses are listed in Supplementary Material S4. In addition, numerous approaches (e.g., GBDTCDA, iCDACMG and SGANRDA) have been proposed to find circRNA-cancer association (Lei and Fang, 2019; Wang L. et al., 2021; Xiao et al., 2021), which will contribute to elucidating the pathogenesis mechanisms and unveiling new insights for tumour diagnosis and targeted therapy. Furthermore, many bioinformatics tools (e.g., Find_circ, CIRI and CIRCexplorer pipelines) have been developed to recognise circRNAs by identifying the back-spliced junction (BSJ) reads (Memczak et al., 2013; Gao et al., 2015; Ma X.-K. et al., 2021). As a novel and increasingly popular research area, the bioinformatics toolboxes for circRNAs discovery and analysis remain in their infancy. The basic work of circRNA research need to be improved, such as establishing high quality dtabases, developing rapid and potent detection tools, and confirming the unified standard for detection methods.
CircRNAs were once considered the waste of error splice; however, recent studies have explored the comprehensive expression patterns of natural circRNAs and then screened and validated them in tumour (Liu et al., 2017). In addition, researchers have designed engineering circRNAs and their regulators for potent and durable protein expression in vitro (Wesselhoeft et al., 2018; Qi et al., 2021a). Artificial circRNAs function as miRNA and protein sponges have been the focus of research attention (Wang Z. et al., 2019; Schreiner et al., 2020). For example, Liu et al. constructed artificial circRNAs, which can suppress gastric carcinoma cell proliferation through sponging miR-21 (Liu X. et al., 2018). Jost et al. proved that artificial circRNAs inhibited viral protein production through sponging miR-122 (Jost et al., 2018). Although artifical circRNAs have many potential applications, they still face challenges due to the immunogenicity (Liu C.-X. et al., 2019). Liu et al. first revealed that synthesised circRNAs without extraneous fragments exhibited minimal immunogenicity and inhibiton related to PKR overreaction (Liu C.-X. et al., 2022). Qu et al. first reported a circRNA vaccine that encodes the trimeric receptor-binding domain of the SARS-CoV-2 spike protein (Qu et al., 2021) and elicits potent neutralizing antibodies and T cell responses, providing robust protection against SARS-CoV-2 (Qu et al., 2022). However, the immunogenicity of in vitro transcription-produced circRNAs is a potential concern and the safety of circRNA vaccines awaits further investigation (Liu L. et al., 2022).
Although great progress has been made in identifying circRNAs, the exact mechanisms of circRNA biogenesis and functions in TC remain largely unexplored. First, does circRNA actually circular? Sun et al. first suggested that circRNAs might not have a simple ring structure but contain a double-stranded structure, thus facilitating circRNAs export to the cytoplasm and making them more easily degraded (Sun et al., 2021). Second, how do circRNA decay? Some circRNAs are degraded by endonucleases (e.g., RNase P) in a primary sequence-dependent manner (Hansen et al., 2011; Park et al., 2019), another mechanisms (e.g., UPF1 and G3BP1) are associated with structure-mediated RNA decay (Liu C.-X. et al., 2019; Fischer et al., 2020). However, the detailed process is largely unknown. It will be essential to elucidate which endoribonuclease opens the closed loop of these circRNAs, how circRNAs are degraded by extracellular or intracellular signals, and what other factors contribute to structure-mediated RNA decay (Guo Y. et al., 2020). Third, extracellular vesicles (EVs) and exosomes have been used as drug and functional RNA delivery vectors in cancer treatment (Yang Z. et al., 2020). EVs-derived RNAs are essential functional cargoes in reciprocal crosstalk within tumor cells and between tumor and stromal cells (Hu W. et al., 2020). In addition, EVs-derived circRNAs can enhance functional recovery in post stroke and may extend the therapeutic window for stroke (Yang L. et al., 2020). However, obstacles that need to be overcome towards clinical utilisation include upscaling of the EVs production and isolation process, and guidelines for appropriate storage (Elsharkasy et al., 2020). Forth, the mechanisms guiding circRNAs exosome assembly, lysosomal exocytosis and endocytosis are poorly understood. Although study clarify that exosomes contain transmembrane and membrane anchoring proteins, which enhance endocytosis (Kamerkar et al., 2017), more efforts are still needed to make the diagnostic and therapeutic potential of exosomes a clinical reality. Finally, knockdown of circFSCN1 and circ_Malat 1 can effectively prevent alloimmune rejection in heart transplantation (Zhang Y. et al., 2018; Wang B. et al., 2021). exosome-based delivery products can induce an early T cell response and initiate antitumor immune responses (Gilligan and Dwyer, 2017; Seo et al., 2018). However, there is no evidence that exosomal circRNAs contribute to preventing immunological rejection in tumour.
Although dysregulated circRNAs and their function contribute to TC initiation and progression, the underlying mechanisms remain poorly defined. First, researchers have proposed that a balance exists between circRNA generation, intracellular localisation, and degradation. Once this balance is tipped, circRNA becomes dysregulated (Li J. et al., 2020). Second, ceRNA hypothesis have been recognised as the most common mechanism for circRNAs to utilise their function, but the function of miRNA sponge still faces challenges (Thomson and Dinger, 2016). Few circRNAs harbour as many miRNA binding sites for a single miRNA as ciRS-7 (Hansen et al., 2013) and circZNF91 (Kristensen et al., 2018), and the abundance of many circRNAs is far lower than that of miRNAs, preventing them from achieving the miRNA sponge effect. In addition to the stoichiometric relevance between the miRNA-binding sites and the mRNA target sites of the miRNA need to be considered, Ago-CLIP/AgoIP and quantitative analysis of specific primers are also required to confirm the function of miRNA sponges. Third, recent study has clarified that the ciRS-7 is upregulated in stromal cells within the tumour microenvironment, but is absent in tumour cells, particularly in classical oncogene-driven adenocarcinomas (Kristensen et al., 2020). The spatial expression patterns of circRNAs at the single-cell level are crucial for understanding the function of circRNAs and advancing the discovery and development of biomarkers in the future. More than fifty clinical trails hve been registered on the website of Chinese Clinical Trial Registry and National Library of Medicine, thus highlight the important roles of circRNAs in human diseases (e.g., pancreatic cancer and COVID-19), but these functions are only the beginning.
Numerous studies investigated various DECs between thyroid tumours and the adjacent non-tumour tissues. Some circRNAs (e.g., hsa_circRNA_047,771) were associated with the BRAFV600E mutation (p < 0.05) in PTC (Ren et al., 2018). The presence of BRAFV600E mutation at PTC diagnosis is associated with aggressive tumour characteristics (p < 0.001) (Xing et al., 2013). Furthermore, BRAFV600E mutation may lead to a decrease in the therapeutic effect of radioactive iodine, resulting in treatment failure or recurrence (Ge et al., 2020). Targeting circRNAs related to BRAFV600E mutation may contribute to reducing the recurrence and improve the outcome of TC. A 5-years cohort study suggested that patients with thyroid nodules increased by ≥ 3 mm in only 8% of patients, and only 3.8% of patients developed nodal metastases (Ito et al., 2014). However, the overtreatment of TC has been recognized as an urgent issue. Many asymptomatic TC patients treated with surgery may suffer from permanent hypoparathyroidism and recurrent laryngeal nerve injuries, and need long-term hormone replacement therapy (Luster et al., 2014; Jegerlehner et al., 2017). Therefore, accurately identifying the circRNAs associated with TC helps in the diagnosis and treatment of TC.
Conclusion
In summary, circRNAs constitute an emerging class of ncRNAs that play crucial roles in the regulation of gene expression by controlling miRNA and protein functions. With the broad applications of high-throughput sequencing technology and bioinformatics analysis in scientific research, the number of circRNAs with known functions is increasing. Notably, circRNAs mediate central biological functions including various physiological and pathophysiological processes, rendering them ideal candidates in the field of cancer research.
Our review discussed and summarised the emerging data and research progress on TC-associated circRNAs, and further highlighted individual circRNAs that may play oncogenic, anticancer, or sensitivity to chemoradiation regulating role in the tumourigenesis, metastasis and therapy resistance of TC by various molecular mechanisms. These circRNAs provide a new area of interest for developing TC diagnostics, prognostics, and therapies. Since the current understanding of circRNAs is basic, much research is required to reveal its regulatory mechanisms and subsequent biological functions in TC.
Author Contributions
Conceptualization of the study was by XY and QZ. Writing and editing were performed by XY, and the manuscript was revised by QZ. Supplementary Material, tables and figures were devised by XY. All the authors read and approved the final manuscript.
Conflict of Interest
The authors declare that the research was conducted in the absence of any commercial or financial relationships that could be construed as a potential conflict of interest.
Publisher’s Note
All claims expressed in this article are solely those of the authors and do not necessarily represent those of their affiliated organizations, or those of the publisher, the editors and the reviewers. Any product that may be evaluated in this article, or claim that may be made by its manufacturer, is not guaranteed or endorsed by the publisher.
Supplementary Material
The Supplementary Material for this article can be found online at: https://www.frontiersin.org/articles/10.3389/fmolb.2022.925389/full#supplementary-material
Supplementary Figure S1 | Network of circRNA-miRNA-mRNA interactions in thyroid cancer.
Supplementary Table S1 | Biological functions and molecular mechanisms of circRNAs in thyroid cancer.
Supplementary Table S2 | Network of circRNA-miRNA-mRNA interactions in thyroid cancer.
Supplementary Table S3 | Databese for circRNA research.
References
Aikins, M. E., Xu, C., and Moon, J. J. (2020). Engineered Nanoparticles for Cancer Vaccination and Immunotherapy. Acc. Chem. Res. 53 (10), 2094–2105. doi:10.1021/acs.accounts.0c00456
Atas, E., Oberhuber, M., and Kenner, L. (2020). The Implications of PDK1-4 on Tumor Energy Metabolism, Aggressiveness and Therapy Resistance. Front. Oncol. 10, 583217. doi:10.3389/fonc.2020.583217
Barrett, S. P., Wang, P. L., and Salzman, J. (2015). Circular RNA Biogenesis Can Proceed through an Exon-Containing Lariat Precursor. Elife 4, e07540. doi:10.7554/eLife.07540
Bi, W., Huang, J., Nie, C., Liu, B., He, G., Han, J., et al. (2018). CircRNA circRNA_102171 Promotes Papillary Thyroid Cancer Progression through Modulating CTNNBIP1-dependent Activation of β-catenin Pathway. J. Exp. Clin. Cancer Res. 37 (1), 275. doi:10.1186/s13046-018-0936-7
Bronisz, A., Rooj, A. K., Krawczyński, K., Peruzzi, P., Salińska, E., Nakano, I., et al. (2020). The Nuclear DICER-Circular RNA Complex Drives the Deregulation of the Glioblastoma Cell microRNAome. Sci. Adv. 6 (51). doi:10.1126/sciadv.abc0221
Chen, F., Feng, Z., Zhu, J., Liu, P., Yang, C., Huang, R., et al. (2018). Emerging Roles of circRNA_NEK6 Targeting miR-370-3p in the Proliferation and Invasion of Thyroid Cancer via Wnt Signaling Pathway. Cancer Biol. Ther. 19 (12), 1139–1152. doi:10.1080/15384047.2018.1480888
Cai, X., Zhao, Z., Dong, J., Lv, Q., Yun, B., Liu, J., et al. (2019). Circular RNA circBACH2 Plays a Role in Papillary Thyroid Carcinoma by Sponging miR-139-5p and Regulating LMO4 Expression. Cell. Death Dis. 10 (3), 184. doi:10.1038/s41419-019-1439-y
Cao, L., Wang, M., Dong, Y., Xu, B., Chen, J., Ding, Y., et al. (2020). Circular RNA circRNF20 Promotes Breast Cancer Tumorigenesis and Warburg Effect through miR-487a/HIF-1α/HK2. Cell. Death Dis. 11 (2), 145. doi:10.1038/s41419-020-2336-0
Capel, B., Swain, A., Nicolis, S., Hacker, A., Walter, M., Koopman, P., et al. (1993). Circular Transcripts of the Testis-Determining Gene Sry in Adult Mouse Testis. Cell. 73 (5), 1019–1030. doi:10.1016/0092-8674(93)90279-y
Cen, J., Liang, Y., Huang, Y., Pan, Y., Shu, G., Zheng, Z., et al. (2021). Circular RNA circSDHC Serves as a Sponge for miR-127-3p to Promote the Proliferation and Metastasis of Renal Cell Carcinoma via the CDKN3/E2F1 axis. Mol. Cancer 20 (1), 19. doi:10.1186/s12943-021-01314-w
Chen, B. J., Huang, S., and Janitz, M. (2019a). Changes in Circular RNA Expression Patterns during Human Foetal Brain Development. Genomics 111 (4), 753–758. doi:10.1016/j.ygeno.2018.04.015
Chen, C., Yu, H., Han, F., Lai, X., Ye, K., Lei, S., et al. (2022a). Tumor-suppressive circRHOBTB3 Is Excreted Out of Cells via Exosome to Sustain Colorectal Cancer Cell Fitness. Mol. Cancer 21 (1), 46. doi:10.1186/s12943-022-01511-1
Chen, F., Yin, S., Feng, Z., Liu, C., Lv, J., Chen, Y., et al. (2021a). Knockdown of circ_NEK6 Decreased 131I Resistance of Differentiated Thyroid Carcinoma via Regulating miR-370-3p/MYH9 Axis. Technol. Cancer Res. Treat. 20, 153303382110049. doi:10.1177/15330338211004950
Chen, H., Li, Q., Yi, R., Li, B., Xiong, D., and Peng, H. (2022b). CircRNA Casein Kinase 1 Gamma 1 (circ‐CSNK1G1) Plays Carcinogenic Effects in Thyroid Cancer by Acting as miR‐149‐5p Sponge and Relieving the Suppression of miR‐149‐5p on Mitogen‐activated Protein Kinase 1 (MAPK1). Clin. Lab. Anal. 36 (2), e24188. doi:10.1002/jcla.24188
Chen, J., Wu, Y., Luo, X., Jin, D., Zhou, W., Ju, Z., et al. (2021b). Circular RNA circRHOBTB3 Represses Metastasis by Regulating the HuR-Mediated mRNA Stability of PTBP1 in Colorectal Cancer. Theranostics 11 (15), 7507–7526. doi:10.7150/thno.59546
Chen, L.-L. (2020). The Expanding Regulatory Mechanisms and Cellular Functions of Circular RNAs. Nat. Rev. Mol. Cell. Biol. 21 (8), 475–490. doi:10.1038/s41580-020-0243-y
Chen, L., Huang, C., and Shan, G. (2022c). Circular RNAs in Physiology and Non-immunological Diseases. Trends Biochem. Sci. 47 (3), 250–264. doi:10.1016/j.tibs.2021.11.004
Chen, L., Wang, C., Sun, H., Wang, J., Liang, Y., Wang, Y., et al. (2021c). The Bioinformatics Toolbox for circRNA Discovery and Analysis. Brief. Bioinform 22 (2), 1706–1728. doi:10.1093/bib/bbaa001
Chen, R.-X., Chen, X., Xia, L.-P., Zhang, J.-X., Pan, Z.-Z., Ma, X.-D., et al. (2019b). N6-methyladenosine Modification of circNSUN2 Facilitates Cytoplasmic Export and Stabilizes HMGA2 to Promote Colorectal Liver Metastasis. Nat. Commun. 10 (1), 4695. doi:10.1038/s41467-019-12651-2
Chen, W., Fu, J., Chen, Y., Li, Y., Ning, L., Huang, D., et al. (2021d). Circular RNA circKIF4A Facilitates the Malignant Progression and Suppresses Ferroptosis by Sponging miR-1231 and Upregulating GPX4 in Papillary Thyroid Cancer. Aging 13 (12), 16500–16512. doi:10.18632/aging.203172
Chen, W., Zhang, T., Bai, Y., Deng, H., Yang, F., Zhu, R., et al. (2021e). Upregulated circRAD18 Promotes Tumor Progression by Reprogramming Glucose Metabolism in Papillary Thyroid Cancer. Gland. Surg. 10 (8), 2500–2510. doi:10.21037/gs-21-481
Chen, X., Han, P., Zhou, T., Guo, X., Song, X., and Li, Y. (2016). circRNADb: A Comprehensive Database for Human Circular RNAs with Protein-Coding Annotations. Sci. Rep. 6, 34985. doi:10.1038/srep34985
Chen, X., Yu, J., Tian, H., Shan, Z., Liu, W., Pan, Z., et al. (2019c). Circle RNA hsa_circRNA_100290 Serves as a ceRNA for miR‐378a to Regulate Oral Squamous Cell Carcinoma Cells Growth via Glucose Transporter‐1 (GLUT1) and Glycolysis. J. Cell. Physiology 234 (11), 19130–19140. doi:10.1002/jcp.28692
Chu, J., Tao, L., Yao, T., Chen, Z., Lu, X., Gao, L., et al. (2021). Circular RNA circRUNX1 Promotes Papillary Thyroid Cancer Progression and Metastasis by Sponging MiR-296-3p and Regulating DDHD2 Expression. Cell. Death Dis. 12 (1), 112. doi:10.1038/s41419-020-03350-8
Conn, S. J., Pillman, K. A., Toubia, J., Conn, V. M., Salmanidis, M., Phillips, C. A., et al. (2015). The RNA Binding Protein Quaking Regulates Formation of circRNAs. Cell. 160 (6), 1125–1134. doi:10.1016/j.cell.2015.02.014
Cooper, D. A., Cortés-López, M., and Miura, P. (2018). Genome-Wide circRNA Profiling from RNA-Seq Data. Methods Mol. Biol. 1724, 27–41. doi:10.1007/978-1-4939-7562-4_3
Cui, W., and Xue, J. (2020). Circular RNA DOCK1 Downregulates microRNA ‐124 to Induce the Growth of Human Thyroid Cancer Cell Lines. Biofactors 46 (4), 591–599. doi:10.1002/biof.1662
Deng, Y., Li, H., Wang, M., Li, N., Tian, T., Wu, Y., et al. (2020). Global Burden of Thyroid Cancer from 1990 to 2017. JAMA Netw. Open 3 (6), e208759. doi:10.1001/jamanetworkopen.2020.8759
Ding, H., Wang, X., Liu, H., and Na, L. (2021a). Higher Circular RNA_0015278 Correlates with Absence of Extrathyroidal Invasion, Lower Pathological Tumor Stages, and Prolonged Disease‐free Survival in Papillary Thyroid Carcinoma Patients. J. Clin. Lab. Anal. 35 (7), e23819. doi:10.1002/jcla.23819
Ding, W., Shi, Y., and Zhang, H. (2021b). Circular RNA circNEURL4 Inhibits Cell Proliferation and Invasion of Papillary Thyroid Carcinoma by Sponging miR-1278 and Regulating LATS1 Expression. Am. J. Transl. Res. 13 (6), 5911–5927.
Dong, L.-P., Chen, L.-Y., Bai, B., Qi, X.-F., Liu, J.-N., and Qin, S. (2022). circ_0067934 Promotes the Progression of Papillary Thyroid Carcinoma Cells through miR-1301-3p/HMGB1 axis. neo 69 (1), 1–15. doi:10.4149/neo_2021_210608N771
Dong, R., Ma, X.-K., Li, G.-W., and Yang, L. (2018). CIRCpedia V2: An Updated Database for Comprehensive Circular RNA Annotation and Expression Comparison. Genomics, Proteomics Bioinforma. 16 (4), 226–233. doi:10.1016/j.gpb.2018.08.001
Du, G., Ma, R., Li, H., He, J., Feng, K., Niu, D., et al. (2021). Increased Expression of Hsa_circ_0002111 and its Clinical Significance in Papillary Thyroid Cancer. Front. Oncol. 11, 644011. doi:10.3389/fonc.2021.644011
Du, W. W., Li, X., Ma, J., Fang, L., Wu, N., Li, F., et al. (2022). Promotion of Tumor Progression by Exosome Transmission of Circular RNA circSKA3. Mol. Ther. - Nucleic Acids 27, 276–292. doi:10.1016/j.omtn.2021.11.027
Du, W. W., Yang, W., Liu, E., Yang, Z., Dhaliwal, P., and Yang, B. B. (2016). Foxo3 Circular RNA Retards Cell Cycle Progression via Forming Ternary Complexes with P21 and CDK2. Nucleic Acids Res. 44 (6), 2846–2858. doi:10.1093/nar/gkw027
Elsharkasy, O. M., Nordin, J. Z., Hagey, D. W., de Jong, O. G., Schiffelers, R. M., Andaloussi, S. E., et al. (2020). Extracellular Vesicles as Drug Delivery Systems: Why and How? Adv. Drug Deliv. Rev. 159, 332–343. doi:10.1016/j.addr.2020.04.004
Enuka, Y., Lauriola, M., Feldman, M. E., Sas-Chen, A., Ulitsky, I., and Yarden, Y. (2016). Circular RNAs Are Long-Lived and Display Only Minimal Early Alterations in Response to a Growth Factor. Nucleic Acids Res. 44 (3), 1370–1383. doi:10.1093/nar/gkv1367
Fan, C., Li, Y., Lan, T., Wang, W., Long, Y., and Yu, S. Y. (2022). Microglia Secrete miR-146a-5p-Containing Exosomes to Regulate Neurogenesis in Depression. Mol. Ther. 30 (3), 1300–1314. doi:10.1016/j.ymthe.2021.11.006
Fan, Y. X., Shi, H. Y., Hu, Y. L., and Jin, X. L. (2020). Circ_0000144 Facilitates the Progression of Thyroid Cancer via the miR‐217/AKT3 Pathway. J. Gene Med. 22 (12), e3269. doi:10.1002/jgm.3269
Feng, J., Chen, W., Dong, X., Wang, J., Mei, X., Deng, J., et al. (2022). CSCD2: an Integrated Interactional Database of Cancer-specific Circular RNAs. Nucleic Acids Res. 50 (D1), D1179–d1183. doi:10.1093/nar/gkab830
Fischer, J. W., Busa, V. F., Shao, Y., and Leung, A. K. L. (2020). Structure-Mediated RNA Decay by UPF1 and G3BP1. Mol. Cell. 78 (1), 70–84.e76. doi:10.1016/j.molcel.2020.01.021
Gao, R., Ye, H., Gao, Q., Wang, N., Zhou, Y., and Duan, H. (2021). Inhibition of Circular RNA_0000285 Prevents Cell Proliferation and Induces Apoptosis in Thyroid Cancer by Sponging microRNA‑654‑3p. Oncol. Lett. 22 (3), 673. doi:10.3892/ol.2021.12934
Gao, W., Guo, H., Niu, M., Zheng, X., Zhang, Y., Xue, X., et al. (2020). circPARD3 Drives Malignant Progression and Chemoresistance of Laryngeal Squamous Cell Carcinoma by Inhibiting Autophagy through the PRKCI-Akt-mTOR Pathway. Mol. Cancer 19 (1), 166. doi:10.1186/s12943-020-01279-2
Gao, Y., Wang, J., and Zhao, F. (2015). CIRI: an Efficient and Unbiased Algorithm for De Novo Circular RNA Identification. Genome Biol. 16 (1), 4. doi:10.1186/s13059-014-0571-3
Ge, J., Wang, J., Wang, H., Jiang, X., Liao, Q., Gong, Q., et al. (2020). The BRAF V600E Mutation Is a Predictor of the Effect of Radioiodine Therapy in Papillary Thyroid Cancer. J. Cancer 11 (4), 932–939. doi:10.7150/jca.33105
Ghosal, S., Das, S., Sen, R., Basak, P., and Chakrabarti, J. (2013). Circ2Traits: a Comprehensive Database for Circular RNA Potentially Associated with Disease and Traits. Front. Genet. 4, 283. doi:10.3389/fgene.2013.00283
Gilligan, K., and Dwyer, R. (2017). Engineering Exosomes for Cancer Therapy. Ijms 18 (6), 1122. doi:10.3390/ijms18061122
Glažar, P., Papavasileiou, P., and Rajewsky, N. (2014). circBase: a Database for Circular RNAs. Rna 20 (11), 1666–1670. doi:10.1261/rna.043687.113
Gong, J., Kong, X., Qi, J., Lu, J., Yuan, S., and Wu, M. (2021). CircRNA_104565 Promoted Cell Proliferation in Papillary Thyroid Carcinoma by Sponging miR-134. Ijgm Vol. 14, 179–185. doi:10.2147/ijgm.S288360
Goodall, G. J., and Wickramasinghe, V. O. (2021). RNA in Cancer. Nat. Rev. Cancer 21 (1), 22–36. doi:10.1038/s41568-020-00306-0
Greene, J., Baird, A.-M., Brady, L., Lim, M., Gray, S. G., McDermott, R., et al. (2017). Circular RNAs: Biogenesis, Function and Role in Human Diseases. Front. Mol. Biosci. 4, 38. doi:10.3389/fmolb.2017.00038
Gu, X., Shi, Y., Dong, M., Jiang, L., Yang, J., and Liu, Z. (2021). Exosomal Transfer of Tumor-Associated Macrophage-Derived Hsa_circ_0001610 Reduces Radiosensitivity in Endometrial Cancer. Cell. Death Dis. 12 (9), 818. doi:10.1038/s41419-021-04087-8
Gui, X., Li, Y., Zhang, X., Su, K., and Cao, W. (2020). Circ_LDLR Promoted the Development of Papillary Thyroid Carcinoma via Regulating miR-195-5p/LIPH axis. Cancer Cell. Int. 20, 241. doi:10.1186/s12935-020-01327-3
Guo, D., Li, F., Zhao, X., Long, B., Zhang, S., Wang, A., et al. (2020a). Circular RNA Expression and Association with the Clinicopathological Characteristics in Papillary Thyroid Carcinoma. Oncol. Rep. 44 (2), 519–532. doi:10.3892/or.2020.7626
Guo, M., Sun, Y., Ding, J., Li, Y., Yang, S., Zhao, Y., et al. (2021). Circular RNA Profiling Reveals a Potential Role of hsa_circ_IPCEF1 in Papillary Thyroid Carcinoma. Mol. Med. Rep. 24 (2). doi:10.3892/mmr.2021.12241
Guo, Y., Wei, X., and Peng, Y. (2020b). Structure-Mediated Degradation of CircRNAs. Trends Cell. Biol. 30 (7), 501–503. doi:10.1016/j.tcb.2020.04.001
Han, J.-y., Guo, S., Wei, N., Xue, R., Li, W., Dong, G., et al. (2020a). ciRS-7 Promotes the Proliferation and Migration of Papillary Thyroid Cancer by Negatively Regulating the miR-7/Epidermal Growth Factor Receptor Axis. BioMed Res. Int. 2020, 1–14. doi:10.1155/2020/9875636
Han, X. T., Jiang, J. Q., Li, M. Z., and Cong, Q. M. (2020b). Circular RNA Circ-ABCB10 Promotes the Proliferation and Invasion of Thyroid Cancer by Targeting KLF6. Eur. Rev. Med. Pharmacol. Sci. 24 (19), 9774. doi:10.26355/eurrev_202010_23170
Hanniford, D., Ulloa-Morales, A., Karz, A., Berzoti-Coelho, M. G., Moubarak, R. S., Sánchez-Sendra, B., et al. (2020). Epigenetic Silencing of CDR1as Drives IGF2BP3-Mediated Melanoma Invasion and Metastasis. Cancer Cell. 37 (1), 55–70.e15. doi:10.1016/j.ccell.2019.12.007
Hansen, T. B., Jensen, T. I., Clausen, B. H., Bramsen, J. B., Finsen, B., Damgaard, C. K., et al. (2013). Natural RNA Circles Function as Efficient microRNA Sponges. Nature 495 (7441), 384–388. doi:10.1038/nature11993
Hansen, T. B., Wiklund, E. D., Bramsen, J. B., Villadsen, S. B., Statham, A. L., Clark, S. J., et al. (2011). miRNA-Dependent Gene Silencing Involving Ago2-Mediated Cleavage of a Circular Antisense RNA. Embo J. 30 (21), 4414–4422. doi:10.1038/emboj.2011.359
He, Y.-D., Tao, W., He, T., Wang, B.-Y., Tang, X.-M., Zhang, L.-M., et al. (2021). A Urine Extracellular Vesicle circRNA Classifier for Detection of High-Grade Prostate Cancer in Patients with Prostate-specific Antigen 2-10 ng/mL at Initial Biopsy. Mol. Cancer 20 (1), 96. doi:10.1186/s12943-021-01388-6
Herzog, B. H., Devarakonda, S., and Govindan, R. (2021). Overcoming Chemotherapy Resistance in SCLC. J. Thorac. Oncol. 16 (12), 2002–2015. doi:10.1016/j.jtho.2021.07.018
Hou, S., Tan, J., Yang, B., He, L., and Zhu, Y. (2018). Effect of Alkylglycerone Phosphate Synthase on the Expression Profile of circRNAs in the Human Thyroid Cancer Cell Line FRO. Oncol. Lett. 15 (5), 7889–7899. doi:10.3892/ol.2018.8356
Hsu, M.-T., and Coca-Prados, M. (1979). Electron Microscopic Evidence for the Circular Form of RNA in the Cytoplasm of Eukaryotic Cells. Nature 280 (5720), 339–340. doi:10.1038/280339a0
Hu, W., Liu, C., Bi, Z.-Y., Zhou, Q., Zhang, H., Li, L.-L., et al. (2020a). Comprehensive Landscape of Extracellular Vesicle-Derived RNAs in Cancer Initiation, Progression, Metastasis and Cancer Immunology. Mol. Cancer 19 (1), 102. doi:10.1186/s12943-020-01199-1
Hu, Z., Zhao, P., Zhang, K., Zang, L., Liao, H., and Ma, W. (2020b). Hsa_circ_0011290 Regulates Proliferation, Apoptosis and Glycolytic Phenotype in Papillary Thyroid Cancer via miR-1252/FSTL1 Signal Pathway. Archives Biochem. Biophysics 685, 108353. doi:10.1016/j.abb.2020.108353
Huang, C., Liang, D., Tatomer, D. C., and Wilusz, J. E. (2018). A Length-dependent Evolutionarily Conserved Pathway Controls Nuclear Export of Circular RNAs. Genes Dev. 32 (9-10), 639–644. doi:10.1101/gad.314856.118
Huang, Y., Xie, Z., Li, X., Chen, W., He, Y., Wu, S., et al. (2021). Development and Validation of a Ferroptosis-Related Prognostic Model for the Prediction of Progression-free Survival and Immune Microenvironment in Patients with Papillary Thyroid Carcinoma. Int. Immunopharmacol. 101 (Pt A), 108156. doi:10.1016/j.intimp.2021.108156
Ito, Y., Miyauchi, A., Kihara, M., Higashiyama, T., Kobayashi, K., and Miya, A. (2014). Patient Age Is Significantly Related to the Progression of Papillary Microcarcinoma of the Thyroid under Observation. Thyroid 24 (1), 27–34. doi:10.1089/thy.2013.0367
Jaaks, P., Coker, E. A., Vis, D. J., Edwards, O., Carpenter, E. F., Leto, S. M., et al. (2022). Effective Drug Combinations in Breast, Colon and Pancreatic Cancer Cells. Nature 603 (7899), 166–173. doi:10.1038/s41586-022-04437-2
Jafari, A., Babajani, A., Abdollahpour-Alitappeh, M., Ahmadi, N., and Rezaei-Tavirani, M. (2021). Exosomes and Cancer: from Molecular Mechanisms to Clinical Applications. Med. Oncol. 38 (4), 45. doi:10.1007/s12032-021-01491-0
Jafari Ghods, F. (2018). Circular RNA in Saliva. Adv. Exp. Med. Biol. 1087, 131–139. doi:10.1007/978-981-13-1426-1_11
Jeck, W. R., and Sharpless, N. E. (2014). Detecting and Characterizing Circular RNAs. Nat. Biotechnol. 32 (5), 453–461. doi:10.1038/nbt.2890
Jeck, W. R., Sorrentino, J. A., Wang, K., Slevin, M. K., Burd, C. E., Liu, J., et al. (2013). Circular RNAs Are Abundant, Conserved, and Associated with ALU Repeats. Rna 19 (2), 141–157. doi:10.1261/rna.035667.112
Jegerlehner, S., Bulliard, J.-L., Aujesky, D., Rodondi, N., Germann, S., Konzelmann, I., et al. (2017). Overdiagnosis and Overtreatment of Thyroid Cancer: A Population-Based Temporal Trend Study. PLoS One 12 (6), e0179387. doi:10.1371/journal.pone.0179387
Jiang, W., Zhang, X., Chu, Q., Lu, S., Zhou, L., Lu, X., et al. (2018). The Circular RNA Profiles of Colorectal Tumor Metastatic Cells. Front. Genet. 9, 34. doi:10.3389/fgene.2018.00034
Jin, X., Wang, Z., Pang, W., Zhou, J., Liang, Y., Yang, J., et al. (2018). Upregulated Hsa_circ_0004458 Contributes to Progression of Papillary Thyroid Carcinoma by Inhibition of miR-885-5p and Activation of RAC1. Med. Sci. Monit. 24, 5488–5500. doi:10.12659/msm.911095
Jost, I., Shalamova, L. A., Gerresheim, G. K., Niepmann, M., Bindereif, A., and Rossbach, O. (2018). Functional Sequestration of microRNA-122 from Hepatitis C Virus by Circular RNA Sponges. RNA Biol. 15 (8), 1–8. doi:10.1080/15476286.2018.1435248
Kalluri, R., and LeBleu, V. S. (2020). The Biology , Function , and Biomedical Applications of Exosomes. Science 367 (6478). doi:10.1126/science.aau6977
Kamerkar, S., LeBleu, V. S., Sugimoto, H., Yang, S., Ruivo, C. F., Melo, S. A., et al. (2017). Exosomes Facilitate Therapeutic Targeting of Oncogenic KRAS in Pancreatic Cancer. Nature 546 (7659), 498–503. doi:10.1038/nature22341
Keutgen, X. M., Sadowski, S. M., and Kebebew, E. (2015). Management of Anaplastic Thyroid Cancer. Gland. Surg. 4 (1), 44–51. doi:10.3978/j.issn.2227-684X.2014.12.02
Kim, J., Gosnell, J. E., and Roman, S. A. (2020). Geographic Influences in the Global Rise of Thyroid Cancer. Nat. Rev. Endocrinol. 16 (1), 17–29. doi:10.1038/s41574-019-0263-x
Kos, A., Dijkema, R., Arnberg, A. C., van der Meide, P. H., and Schellekens, H. (1986). The Hepatitis Delta (δ) Virus Possesses a Circular RNA. Nature 323 (6088), 558–560. doi:10.1038/323558a0
Kristensen, L. S., Ebbesen, K. K., Sokol, M., Jakobsen, T., Korsgaard, U., Eriksen, A. C., et al. (2020). Spatial Expression Analyses of the Putative Oncogene ciRS-7 in Cancer Reshape the microRNA Sponge Theory. Nat. Commun. 11 (1), 4551. doi:10.1038/s41467-020-18355-2
Kristensen, L. S., Okholm, T. L. H., Venø, M. T., and Kjems, J. (2018). Circular RNAs Are Abundantly Expressed and Upregulated during Human Epidermal Stem Cell Differentiation. RNA Biol. 15 (2), 280–291. doi:10.1080/15476286.2017.1409931
Li, Z., Xu, J., Guan, H., Lai, J., Yang, X., and Ma, J. (2022). Circ_0059354 Aggravates the Progression of Papillary Thyroid Carcinoma by Elevating ARFGEF1 through Sponging miR-766-3p. J. Endocrinol. Invest. 45 (4), 825–836. doi:10.1007/s40618-021-01713-2
Lampropoulou, D. I., Pliakou, E., Aravantinos, G., Filippou, D., and Gazouli, M. (2022). The Role of Exosomal Non-coding RNAs in Colorectal Cancer Drug Resistance. Ijms 23 (3), 1473. doi:10.3390/ijms23031473
Lan, X., Cao, J., Xu, J., Chen, C., Zheng, C., Wang, J., et al. (2018a). Decreased Expression of Hsa_circ_0137287 Predicts Aggressive Clinicopathologic Characteristics in Papillary Thyroid Carcinoma. J. Clin. Lab. Anal. 32 (8), e22573. doi:10.1002/jcla.22573
Lan, X., Xu, J., Chen, C., Zheng, C., Wang, J., Cao, J., et al. (2018b). The Landscape of Circular RNA Expression Profiles in Papillary Thyroid Carcinoma Based on RNA Sequencing. Cell. Physiol. Biochem. 47 (3), 1122–1132. doi:10.1159/000490188
Legnini, I., Di Timoteo, G., Rossi, F., Morlando, M., Briganti, F., Sthandier, O., et al. (2017). Circ-ZNF609 Is a Circular RNA that Can Be Translated and Functions in Myogenesis. Mol. Cell. 66 (1), 22–37.e29. doi:10.1016/j.molcel.2017.02.017
Lei, X., and Fang, Z. (2019). GBDTCDA: Predicting circRNA-Disease Associations Based on Gradient Boosting Decision Tree with Multiple Biological Data Fusion. Int. J. Biol. Sci. 15 (13), 2911–2924. doi:10.7150/ijbs.33806
Li, C., Zhu, L., Fu, L., Han, M., Li, Y., Meng, Z., et al. (2021a). CircRNA NRIP1 Promotes Papillary Thyroid Carcinoma Progression by Sponging Mir-195-5p and Modulating the P38 MAPK and JAK/STAT Pathways. Diagn Pathol. 16 (1), 93. doi:10.1186/s13000-021-01153-9
Li, J., Sun, D., Pu, W., Wang, J., and Peng, Y. (2020a). Circular RNAs in Cancer: Biogenesis, Function, and Clinical Significance. Trends Cancer 6 (4), 319–336. doi:10.1016/j.trecan.2020.01.012
Li, S., Li, Y., Chen, B., Zhao, J., Yu, S., Tang, Y., et al. (2018a). exoRBase: a Database of circRNA, lncRNA and mRNA in Human Blood Exosomes. Nucleic Acids Res. 46 (D1), D106–d112. doi:10.1093/nar/gkx891
Li, S., Yang, J., Liu, X., Guo, R., and Zhang, R. (2020b). circITGA7 Functions as an Oncogene by Sponging miR-198 and Upregulating FGFR1 Expression in Thyroid Cancer. BioMed Res. Int. 2020, 1–8. doi:10.1155/2020/8084028
Li, X., Liu, C.-X., Xue, W., Zhang, Y., Jiang, S., Yin, Q.-F., et al. (2017). Coordinated circRNA Biogenesis and Function with NF90/NF110 in Viral Infection. Mol. Cell. 67 (2), 214–227.e217. doi:10.1016/j.molcel.2017.05.023
Li, X., Tian, Y., Hu, Y., Yang, Z., Zhang, L., and Luo, J. (2018b). CircNUP214 Sponges miR-145 to Promote the Expression of ZEB2 in Thyroid Cancer Cells. Biochem. Biophysical Res. Commun. 507 (1-4), 168–172. doi:10.1016/j.bbrc.2018.10.200
Li, X., Yang, L., and Chen, L.-L. (2018c). The Biogenesis, Functions, and Challenges of Circular RNAs. Mol. Cell. 71 (3), 428–442. doi:10.1016/j.molcel.2018.06.034
Li, X., Yang, S., Zhao, C., Yang, J., Li, C., Shen, W., et al. (2021b). CircHACE1 Functions as a Competitive Endogenous RNA to Curb Differentiated Thyroid Cancer Progression by Upregulating Tfcp2L1 through Adsorbing miR-346. Endocr. J. 68 (8), 1011–1025. doi:10.1507/endocrj.EJ20-0806
Li, X., Zhang, J.-L., Lei, Y.-N., Liu, X.-Q., Xue, W., Zhang, Y., et al. (2021c). Linking Circular Intronic RNA Degradation and Function in Transcription by RNase H1. Sci. China Life Sci. 64 (11), 1795–1809. doi:10.1007/s11427-021-1993-6
Li, Y., Qin, J., He, Z., Cui, G., Zhang, K., and Wu, B. (2021d). Knockdown of circPUM1 Impedes Cell Growth, Metastasis and Glycolysis of Papillary Thyroid Cancer via Enhancing MAPK1 Expression by Serving as the Sponge of miR-21-5p. Genes Genom 43 (2), 141–150. doi:10.1007/s13258-020-01023-6
Li, Y., Zheng, Q., Bao, C., Li, S., Guo, W., Zhao, J., et al. (2015a). Circular RNA Is Enriched and Stable in Exosomes: a Promising Biomarker for Cancer Diagnosis. Cell. Res. 25 (8), 981–984. doi:10.1038/cr.2015.82
Li, Z., Huang, C., Bao, C., Chen, L., Lin, M., Wang, X., et al. (2015b). Exon-intron Circular RNAs Regulate Transcription in the Nucleus. Nat. Struct. Mol. Biol. 22 (3), 256–264. doi:10.1038/nsmb.2959
Li, Z., Huang, X., Liu, A., Xu, J., Lai, J., Guan, H., et al. (2021e). Circ_PSD3 Promotes the Progression of Papillary Thyroid Carcinoma via the miR-637/HEMGN axis. Life Sci. 264, 118622. doi:10.1016/j.lfs.2020.118622
Li, Z., Xu, J., Guan, H., Lai, J., Yang, X., and Ma, J. (2021f). Circ_0059354 Aggravates the Progression of Papillary Thyroid Carcinoma by Elevating ARFGEF1 through Sponging miR-766-3p. J. Endocrinol. Invest. 45, 825–836. doi:10.1007/s40618-021-01713-2
Lin, Q., Qi, Q., Hou, S., Chen, Z., Jiang, N., Zhang, L., et al. (2021). Exosomal Circular RNA Hsa_circ_007293 Promotes Proliferation, Migration, Invasion, and Epithelial-Mesenchymal Transition of Papillary Thyroid Carcinoma Cells through Regulation of the microRNA-653-5p/paired Box 6 axis. Bioengineered 12 (2), 10136–10149. doi:10.1080/21655979.2021.2000745
Liu, C.-X., Guo, S.-K., Nan, F., Xu, Y.-F., Yang, L., and Chen, L.-L. (2022a). RNA Circles with Minimized Immunogenicity as Potent PKR Inhibitors. Mol. Cell. 82 (2), 420–434.e426. doi:10.1016/j.molcel.2021.11.019
Liu, C.-X., Li, X., Nan, F., Jiang, S., Gao, X., Guo, S.-K., et al. (2019a). Structure and Degradation of Circular RNAs Regulate PKR Activation in Innate Immunity. Cell. 177 (4), 865–880.e821. doi:10.1016/j.cell.2019.03.046
Liu, F., Zhang, J., Qin, L., Yang, Z., Xiong, J., Zhang, Y., et al. (2018a). Circular RNA EIF6 (Hsa_circ_0060060) Sponges miR-144-3p to Promote the Cisplatin-Resistance of Human Thyroid Carcinoma Cells by Autophagy Regulation. Aging 10 (12), 3806–3820. doi:10.18632/aging.101674
Liu, J., Li, H., Wei, C., Ding, J., Lu, J., Pan, G., et al. (2020a). circFAT1(e2) Promotes Papillary Thyroid Cancer Proliferation, Migration, and Invasion via the miRNA-873/ZEB1 Axis. Comput. Math. Methods Med. 2020, 1–9. doi:10.1155/2020/1459368
Liu, J., Liu, T., Wang, X., and He, A. (2017). Circles Reshaping the RNA World: from Waste to Treasure. Mol. Cancer 16 (1), 58. doi:10.1186/s12943-017-0630-y
Liu, J., Zheng, X., and Liu, H. (2020b). Hsa_circ_0102272 Serves as a Prognostic Biomarker and Regulates Proliferation, Migration and Apoptosis in Thyroid Cancer. J. Gene Med. 22 (9), e3209. doi:10.1002/jgm.3209
Liu, L., Iketani, S., Guo, Y., Chan, J. F.-W., Wang, M., Liu, L., et al. (2022b). Striking Antibody Evasion Manifested by the Omicron Variant of SARS-CoV-2. Nature 602 (7898), 676–681. doi:10.1038/s41586-021-04388-0
Liu, L., Yan, C., Tao, S., and Wang, H. (2020c). Circ_0058124 Aggravates the Progression of Papillary Thyroid Carcinoma by Activating LMO4 Expression via Targeting miR-370-3p. Cmar Vol. 12, 9459–9470. doi:10.2147/cmar.S271778
Liu, M., Wang, Q., Shen, J., Yang, B. B., and Ding, X. (2019b). Circbank: a Comprehensive Database for circRNA with Standard Nomenclature. RNA Biol. 16 (7), 899–905. doi:10.1080/15476286.2019.1600395
Liu, Q.-P., Ge, P., Wang, Q.-N., Zhang, S.-Y., Yang, Y.-Q., Lv, M.-Q., et al. (2021a). Circular RNA-CDR1as Is Involved in Lung Injury Induced by Long-Term Formaldehyde Inhalation. Inhal. Toxicol. 33 (9-14), 325–333. doi:10.1080/08958378.2021.1999350
Liu, Q., Pan, L.-z., Hu, M., and Ma, J.-y. (2020d). Molecular Network-Based Identification of Circular RNA-Associated ceRNA Network in Papillary Thyroid Cancer. Pathol. Oncol. Res. 26 (2), 1293–1299. doi:10.1007/s12253-019-00697-y
Liu, W., Zhao, J., Jin, M., and Zhou, M. (2019c). circRAPGEF5 Contributes to Papillary Thyroid Proliferation and Metastatis by Regulation miR-198/FGFR1. Mol. Ther. - Nucleic Acids 14, 609–616. doi:10.1016/j.omtn.2019.01.003
Liu, X., Abraham, J. M., Cheng, Y., Wang, Z., Wang, Z., Zhang, G., et al. (2018b). Synthetic Circular RNA Functions as a miR-21 Sponge to Suppress Gastric Carcinoma Cell Proliferation. Mol. Ther. - Nucleic Acids 13, 312–321. doi:10.1016/j.omtn.2018.09.010
Liu, X., Wang, X., Li, J., Hu, S., Deng, Y., Yin, H., et al. (2020e). Identification of mecciRNAs and Their Roles in the Mitochondrial Entry of Proteins. Sci. China Life Sci. 63 (10), 1429–1449. doi:10.1007/s11427-020-1631-9
Liu, Y.-C., Li, J.-R., Sun, C.-H., Andrews, E., Chao, R.-F., Lin, F.-M., et al. (2016). CircNet: a Database of Circular RNAs Derived from Transcriptome Sequencing Data. Nucleic Acids Res. 44 (D1), D209–D215. doi:10.1093/nar/gkv940
Liu, Y., Chen, G., Wang, B., Wu, H., Zhang, Y., and Ye, H. (2021b). Silencing circRNA Protein Kinase C Iota (Circ-PRKCI) Suppresses Cell Progression and Glycolysis of Human Papillary Thyroid Cancer through Circ-PRKCI/miR-335/e2f3 ceRNA axis. Endocr. J. 68 (6), 713–727. doi:10.1507/endocrj.EJ20-0726
Long, M. Y., Chen, J. W., Zhu, Y., Luo, D. Y., Lin, S. J., Peng, X. Z., et al. (2020). Comprehensive Circular RNA Profiling Reveals the Regulatory Role of circRNA_0007694 in Papillary Thyroid Carcinoma. Am. J. Transl. Res. 12 (4), 1362–1378.
Lou, W., Ding, B., Wang, J., and Xu, Y. (2020). The Involvement of the Hsa_circ_0088494-miR-876-3p-Ctnnb1/ccnd1 Axis in Carcinogenesis and Progression of Papillary Thyroid Carcinoma. Front. Cell. Dev. Biol. 8, 605940. doi:10.3389/fcell.2020.605940
Luan, S., Fu, P., Wang, X., Gao, Y., Shi, K., and Guo, Y. (2020). Circular RNA Circ-NCOR2 Accelerates Papillary Thyroid Cancer Progression by Sponging miR-516a-5p to Upregulate Metastasis-Associated Protein 2 Expression. J. Int. Med. Res. 48 (9), 030006052093465. doi:10.1177/0300060520934659
Luo, Q., Guo, F., Fu, Q., and Sui, G. (2021). hsa_circ_0001018 Promotes Papillary Thyroid Cancer by Facilitating Cell Survival, Invasion, G1/S Cell Cycle Progression, and Repressing Cell Apoptosis via Crosstalk with miR-338-3p and SOX4. Mol. Ther. - Nucleic Acids 24, 591–609. doi:10.1016/j.omtn.2021.02.023
Luster, M., Weber, T., and Verburg, F. A. (2014). Differentiated Thyroid Cancer-Personalized Therapies to Prevent Overtreatment. Nat. Rev. Endocrinol. 10 (9), 563–574. doi:10.1038/nrendo.2014.100
Lv, C., Sun, W., Huang, J., Qin, Y., Ji, X., and Zhang, H. (2021). Expression Profiles of Circular RNAs in Human Papillary Thyroid Carcinoma Based on RNA Deep Sequencing. Ott Vol. 14, 3821–3832. doi:10.2147/ott.S316292
Ma, J., and Kan, Z. (2021). Circular RNA Circ_0008274 Enhances the Malignant Progression of Papillary Thyroid Carcinoma via Modulating Solute Carrier Family 7 Member 11 by Sponging miR-154-3p. Endocr. J. 68 (5), 543–552. doi:10.1507/endocrj.EJ20-0453
Ma, W., Zhao, P., Zang, L., Zhang, K., Liao, H., and Hu, Z. (2021a). CircTP53 Promotes the Proliferation of Thyroid Cancer via Targeting miR-1233-3p/MDM2 axis. J. Endocrinol. Invest. 44 (2), 353–362. doi:10.1007/s40618-020-01317-2
Ma, X.-K., Xue, W., Chen, L.-L., and Yang, L. (2021b). CIRCexplorer Pipelines for circRNA Annotation and Quantification from Non-polyadenylated RNA-Seq Datasets. Methods 196, 3–10. doi:10.1016/j.ymeth.2021.02.008
Mao, Y., Huo, Y., Li, J., Zhao, Y., Wang, Y., Sun, L., et al. (2021). circRPS28 (Hsa_circ_0049055) Is a Novel Contributor for Papillary Thyroid Carcinoma by Regulating Cell Growth and Motility via Functioning as ceRNA for miR-345-5p to Regulate Frizzled Family Receptor 8 (FZD8). Endocr. J. 68 (11), 1267–1281. doi:10.1507/endocrj.EJ21-0072
Memczak, S., Jens, M., Elefsinioti, A., Torti, F., Krueger, J., Rybak, A., et al. (2013). Circular RNAs Are a Large Class of Animal RNAs with Regulatory Potency. Nature 495 (7441), 333–338. doi:10.1038/nature11928
Memczak, S., Papavasileiou, P., Peters, O., and Rajewsky, N. (2015). Identification and Characterization of Circular RNAs as a New Class of Putative Biomarkers in Human Blood. PLoS One 10 (10), e0141214. doi:10.1371/journal.pone.0141214
Meng, X., Hu, D., Zhang, P., Chen, Q., and Chen, M. (20192019). CircFunBase: A Database for Functional Circular RNAs. Oxford: Database. doi:10.1093/database/baz003
Nie, C., Han, J., Bi, W., Qiu, Z., Chen, L., Yu, J., et al. (2022). Circular RNA Circ_0000644 Promotes Papillary Thyroid Cancer Progression via Sponging miR-1205 and Regulating E2F3 Expression. Cell. Cycle 21 (2), 126–139. doi:10.1080/15384101.2021.2012334
Okholm, T. L. H., Sathe, S., Park, S. S., Kamstrup, A. B., Rasmussen, A. M., Shankar, A., et al. (2020). Transcriptome-wide Profiles of Circular RNA and RNA-Binding Protein Interactions Reveal Effects on Circular RNA Biogenesis and Cancer Pathway Expression. Genome Med. 12 (1), 112. doi:10.1186/s13073-020-00812-8
Pan, Y., Xu, T., Liu, Y., Li, W., and Zhang, W. (2019). Upregulated Circular RNA Circ_0025033 Promotes Papillary Thyroid Cancer Cell Proliferation and Invasion via Sponging miR-1231 and miR-1304. Biochem. Biophysical Res. Commun. 510 (2), 334–338. doi:10.1016/j.bbrc.2019.01.108
Park, O. H., Ha, H., Lee, Y., Boo, S. H., Kwon, D. H., Song, H. K., et al. (2019). Endoribonucleolytic Cleavage of m6A-Containing RNAs by RNase P/MRP Complex. Mol. Cell. 74 (3), 494–507.e498. doi:10.1016/j.molcel.2019.02.034
Patop, I. L., Wüst, S., and Kadener, S. (2019). Past, Present, and Future of Circ RNA S. Embo J. 38 (16), e100836. doi:10.15252/embj.2018100836
Peng, N., Shi, L., Zhang, Q., Hu, Y., Wang, N., and Ye, H. (2017). Microarray Profiling of Circular RNAs in Human Papillary Thyroid Carcinoma. PLoS One 12 (3), e0170287. doi:10.1371/journal.pone.0170287
Piwecka, M., Glažar, P., Hernandez-Miranda, L. R., Memczak, S., Wolf, S. A., Rybak-Wolf, A., et al. (2017). Loss of a Mammalian Circular RNA Locus Causes miRNA Deregulation and Affects Brain Function. Science 357 (6357). doi:10.1126/science.aam8526
Pu, W., Shi, X., Yu, P., Zhang, M., Liu, Z., Tan, L., et al. (2021). Single-cell Transcriptomic Analysis of the Tumor Ecosystems Underlying Initiation and Progression of Papillary Thyroid Carcinoma. Nat. Commun. 12 (1), 6058. doi:10.1038/s41467-021-26343-3
Qi, Y., Han, W., Chen, D., Zhao, J., Bai, L., Huang, F., et al. (2021a). Engineering Circular RNA Regulators to Specifically Promote Circular RNA Production. Theranostics 11 (15), 7322–7336. doi:10.7150/thno.56990
Qi, Y., He, J., Zhang, Y., Wang, L., Yu, Y., Yao, B., et al. (2021b). Circular RNA Hsa_circ_0001666 Sponges miR‑330‑5p, miR‑193a‑5p and miR‑326, and Promotes Papillary Thyroid Carcinoma Progression via Upregulation of ETV4. Oncol. Rep. 45 (4). doi:10.3892/or.2021.8001
Qiu, J., Sun, M., Sun, M., Zang, C., Jiang, L., Qin, Z., et al. (2021). Five Genes Involved in Circular RNA-Associated Competitive Endogenous RNA Network Correlates with Metastasis in Papillary Thyroid Carcinoma. Mbe 18 (6), 9016–9032. doi:10.3934/mbe.2021444
Qu, L., Yi, Z., Shen, Y., Lin, L., Chen, F., Xu, Y., et al. (2021). Circular RNA Vaccines against SARS-CoV-2 and Emerging Variants. bioRxiv, 435594. 2021.2003.2016. doi:10.1101/2021.03.16.435594
Qu, L., Yi, Z., Shen, Y., Lin, L., Chen, F., Xu, Y., et al. (2022). Circular RNA Vaccines against SARS-CoV-2 and Emerging Variants. Cell. 185 (10), 1728–1744.e1716. doi:10.1016/j.cell.2022.03.044
Ren, H., Liu, Z., Liu, S., Zhou, X., Wang, H., Xu, J., et al. (2018). Profile and Clinical Implication of Circular RNAs in Human Papillary Thyroid Carcinoma. PeerJ 6, e5363. doi:10.7717/peerj.5363
Ren, H., Song, Z., Chao, C., and Mao, W. (2021). circCCDC66 Promotes Thyroid Cancer Cell Proliferation, Migratory and Invasive Abilities and Glycolysis through the miR‑211‑5p/PDK4 axis. Oncol. Lett. 21 (5), 416. doi:10.3892/ol.2021.12677
Röhrig, F., and Schulze, A. (2016). The Multifaceted Roles of Fatty Acid Synthesis in Cancer. Nat. Rev. Cancer 16 (11), 732–749. doi:10.1038/nrc.2016.89
Salzman, J., Gawad, C., Wang, P. L., Lacayo, N., and Brown, P. O. (2012). Circular RNAs Are the Predominant Transcript Isoform from Hundreds of Human Genes in Diverse Cell Types. PLoS One 7 (2), e30733. doi:10.1371/journal.pone.0030733
Sa, R., Guo, M., Liu, D., and Guan, F. (2021). AhR Antagonist Promotes Differentiation of Papillary Thyroid Cancer via Regulating circSH2B3/miR-4640-5P/IGF2BP2 Axis. Front. Pharmacol. 12, 795386. doi:10.3389/fphar.2021.795386
Sanger, H. L., Klotz, G., Riesner, D., Gross, H. J., and Kleinschmidt, A. K. (1976). Viroids Are Single-Stranded Covalently Closed Circular RNA Molecules Existing as Highly Base-Paired Rod-like Structures. Proc. Natl. Acad. Sci. U.S.A. 73 (11), 3852–3856. doi:10.1073/pnas.73.11.3852
Schreiner, S., Didio, A., Hung, L.-H., and Bindereif, A. (2020). Design and Application of Circular RNAs with Protein-Sponge Function. Nucleic Acids Res. 48 (21), 12326–12335. doi:10.1093/nar/gkaa1085
Seimiya, T., Otsuka, M., Iwata, T., Shibata, C., Tanaka, E., Suzuki, T., et al. (2020). Emerging Roles of Exosomal Circular RNAs in Cancer. Front. Cell. Dev. Biol. 8, 568366. doi:10.3389/fcell.2020.568366
Seo, N., Akiyoshi, K., and Shiku, H. (2018). Exosome‐mediated Regulation of Tumor Immunology. Cancer Sci. 109 (10), 2998–3004. doi:10.1111/cas.13735
Shi, E., Ye, J., Zhang, R., Ye, S., Zhang, S., Wang, Y., et al. (2020). A Combination of circRNAs as a Diagnostic Tool for Discrimination of Papillary Thyroid Cancer. Ott Vol. 13, 4365–4372. doi:10.2147/ott.S247796
Shi, P., Liu, Y., Yang, D., Wu, Y., Zhang, L., Jing, S., et al. (2022). CircRNA ZNF609 Promotes the Growth and Metastasis of Thyroid Cancer In Vivo and In Vitro by Downregulating miR-514a-5p. Bioengineered 13 (2), 4372–4384. doi:10.1080/21655979.2022.2033015
Shu, T., Yang, L., Sun, L., Lu, J., and Zhan, X. (2020). CircHIPK3 Promotes Thyroid Cancer Tumorigenesis and Invasion through the Mirna-338-3p/RAB23 Axis. Med. Princ. Pract. doi:10.1159/000512548
Stine, Z. E., Schug, Z. T., Salvino, J. M., and Dang, C. V. (2022). Targeting Cancer Metabolism in the Era of Precision Oncology. Nat. Rev. Drug Discov. 21 (2), 141–162. doi:10.1038/s41573-021-00339-6
Stone, R. M., Mandrekar, S. J., Sanford, B. L., Laumann, K., Geyer, S., Bloomfield, C. D., et al. (2017). Midostaurin Plus Chemotherapy for Acute Myeloid Leukemia with aFLT3Mutation. N. Engl. J. Med. 377 (5), 454–464. doi:10.1056/NEJMoa1614359
Sun, D., Chen, L., Lv, H., Gao, Y., Liu, X., and Zhang, X. (2020a). Circ_0058124 Upregulates MAPK1 Expression to Promote Proliferation, Metastasis and Metabolic Abilities in Thyroid Cancer through Sponging miR-940. Ott Vol. 13, 1569–1581. doi:10.2147/ott.S237307
Sun, H., Wu, Z., Liu, M., Yu, L., Li, J., Zhang, J., et al. (2021). CircRNA May Not Be "Circular". Front. Genet. 12, 633750. doi:10.3389/fgene.2021.633750
Sun, J. W., Qiu, S., Yang, J. Y., Chen, X., and Li, H. X. (2020b). Hsa_circ_0124055 and Hsa_circ_0101622 Regulate Proliferation and Apoptosis in Thyroid Cancer and Serve as Prognostic and Diagnostic Indicators. Eur. Rev. Med. Pharmacol. Sci. 24 (8), 4348–4360. doi:10.26355/eurrev_202004_21016
Sung, H., Ferlay, J., Siegel, R. L., Laversanne, M., Soerjomataram, I., Jemal, A., et al. (2021). Global Cancer Statistics 2020: GLOBOCAN Estimates of Incidence and Mortality Worldwide for 36 Cancers in 185 Countries. CA A Cancer J. Clin. 71 (3), 209–249. doi:10.3322/caac.21660
Suzuki, H., and Tsukahara, T. (2014). A View of Pre-mRNA Splicing from RNase R Resistant RNAs. Ijms 15 (6), 9331–9342. doi:10.3390/ijms15069331
Tang, Z., Li, X., Zhao, J., Qian, F., Feng, C., Li, Y., et al. (2019). TRCirc: a Resource for Transcriptional Regulation Information of circRNAs. Brief. Bioinform 20 (6), 2327–2333. doi:10.1093/bib/bby083
Tatomer, D. C., Liang, D., and Wilusz, J. E. (2017). Inducible Expression of Eukaryotic Circular RNAs from Plasmids. Methods Mol. Biol. 1648, 143–154. doi:10.1007/978-1-4939-7204-3_11
Thomson, D. W., and Dinger, M. E. (2016). Endogenous microRNA Sponges: Evidence and Controversy. Nat. Rev. Genet. 17 (5), 272–283. doi:10.1038/nrg.2016.20
Trouttet-Masson, S., Selmi-Ruby, S., Bernier-Valentin, F., Porra, V., Berger-Dutrieux, N., Decaussin, M., et al. (2004). Evidence for Transcriptional and Posttranscriptional Alterations of the Sodium/iodide Symporter Expression in Hypofunctioning Benign and Malignant Thyroid Tumors. Am. J. Pathology 165 (1), 25–34. doi:10.1016/s0002-9440(10)63272-5
Tsitsipatis, D., Grammatikakis, I., Driscoll, R. K., Yang, X., Abdelmohsen, K., Harris, S. C., et al. (2021). AUF1 Ligand circPCNX Reduces Cell Proliferation by Competing with P21 mRNA to Increase P21 Production. Nucleic Acids Res. 49 (3), 1631–1646. doi:10.1093/nar/gkaa1246
Tuttle, R. M. (2018). Controversial Issues in Thyroid Cancer Management. J. Nucl. Med. 59 (8), 1187–1194. doi:10.2967/jnumed.117.192559
van Zonneveld, A. J., Kölling, M., Bijkerk, R., and Lorenzen, J. M. (2021). Circular RNAs in Kidney Disease and Cancer. Nat. Rev. Nephrol. 17 (12), 814–826. doi:10.1038/s41581-021-00465-9
Vo, J. N., Cieslik, M., Zhang, Y., Shukla, S., Xiao, L., Zhang, Y., et al. (2019). The Landscape of Circular RNA in Cancer. Cell. 176 (4), 869–881.e813. doi:10.1016/j.cell.2018.12.021
Wang, B., Zhou, Q., Li, A., Li, S., Greasley, A., Skaro, A., et al. (2021a). Preventing Alloimmune Rejection Using Circular RNA FSCN1-Silenced Dendritic Cells in Heart Transplantation. J. Heart Lung Transplant. 40 (7), 584–594. doi:10.1016/j.healun.2021.03.025
Wang, H.-H., Ma, J.-N., and Zhan, X.-R. (2021b). Circular RNA Circ_0067934 Attenuates Ferroptosis of Thyroid Cancer Cells by miR-545-3p/SLC7A11 Signaling. Front. Endocrinol. 12, 670031. doi:10.3389/fendo.2021.670031
Wang, H., Yan, X., Zhang, H., and Zhan, X. (2019a). CircRNA Circ_0067934 Overexpression Correlates with Poor Prognosis and Promotes Thyroid Carcinoma Progression. Med. Sci. Monit. 25, 1342–1349. doi:10.12659/msm.913463
Wang, J., Yu, F., Shang, Y., Ping, Z., and Liu, L. (2020a). Thyroid Cancer: Incidence and Mortality Trends in China, 2005-2015. Endocrine 68 (1), 163–173. doi:10.1007/s12020-020-02207-6
Wang, L., Luo, T., Bao, Z., Li, Y., and Bu, W. (2018a). Intrathecal circHIPK3 shRNA Alleviates Neuropathic Pain in Diabetic Rats. Biochem. Biophysical Res. Commun. 505 (3), 644–650. doi:10.1016/j.bbrc.2018.09.158
Wang, L., Wang, W., Cai, Y., Zhou, Y., Jiang, J., Ning, Y., et al. (2022a). Circ-NUP214 Promotes Papillary Thyroid Carcinoma Tumorigenesis by Regulating HK2 Expression through miR-15a-5p. Biochem. Genet. doi:10.1007/s10528-022-10192-w
Wang, L., Yan, X., You, Z.-H., Zhou, X., Li, H.-Y., and Huang, Y.-A. (2021c). SGANRDA: Semi-supervised Generative Adversarial Networks for Predicting circRNA-Disease Associations. Brief. Bioinform 22 (5). doi:10.1093/bib/bbab028
Wang, L. Y., Palmer, F. L., Nixon, I. J., Thomas, D., Patel, S. G., Shaha, A. R., et al. (2014). Multi-organ Distant Metastases Confer Worse Disease-specific Survival in Differentiated Thyroid Cancer. Thyroid 24 (11), 1594–1599. doi:10.1089/thy.2014.0173
Wang, M., Chen, B., Ru, Z., and Cong, L. (2018b). CircRNA Circ-ITCH Suppresses Papillary Thyroid Cancer Progression through miR-22-3p/CBL/β-catenin Pathway. Biochem. Biophysical Res. Commun. 504 (1), 283–288. doi:10.1016/j.bbrc.2018.08.175
Wang, W., Huang, C., Luo, P., Yao, J., Li, J., Wang, W., et al. (2021d). Circular RNA circWDR27 Promotes Papillary Thyroid Cancer Progression by Regulating miR-215-5p/TRIM44 Axis. Ott Vol. 14, 3281–3293. doi:10.2147/ott.S290270
Wang, Y. F., Li, M. Y., Tang, Y. F., Jia, M., Liu, Z., and Li, H. Q. (2020b). Circular RNA circEIF3I Promotes Papillary Thyroid Carcinoma Progression through Competitively Binding to miR-149 and Upregulating KIF2A Expression. Am. J. Cancer Res. 10 (4), 1130–1139.
Wang, Z., Ma, K., Cheng, Y., Abraham, J. M., Liu, X., Ke, X., et al. (2019b). Synthetic Circular Multi-miR Sponge Simultaneously Inhibits miR-21 and miR-93 in Esophageal Carcinoma. Lab. Invest. 99 (10), 1442–1453. doi:10.1038/s41374-019-0273-2
Wang, Z., Yu, R., Chen, X., Bao, H., Cao, R., Li, A.-N., et al. (2022b). Clinical Utility of Cerebrospinal Fluid-Derived Circular RNAs in Lung Adenocarcinoma Patients with Brain Metastases. J. Transl. Med. 20 (1), 74. doi:10.1186/s12967-022-03274-1
Warburg, O., Wind, F., and Negelein, E. (1927). The Metabolism of Tumors in the Body. J. Gen. Physiol. 8 (6), 519–530. doi:10.1085/jgp.8.6.519
Wei, H., Pan, L., Tao, D., and Li, R. (2018). Circular RNA circZFR Contributes to Papillary Thyroid Cancer Cell Proliferation and Invasion by Sponging miR-1261 and Facilitating C8orf4 Expression. Biochem. Biophysical Res. Commun. 503 (1), 56–61. doi:10.1016/j.bbrc.2018.05.174
Wen, S., Luo, Y., Wu, W., Zhang, T., Yang, Y., Ji, Q., et al. (2021a). Identification of Lipid Metabolism-Related Genes as Prognostic Indicators in Papillary Thyroid Cancer. Acta Biochim. Biophys. Sin. (Shanghai) 53 (12), 1579–1589. doi:10.1093/abbs/gmab145
Wen, X., Du, J., and Wang, X. (2021b). Circ_0039411 Promotes Papillary Thyroid Carcinoma Development through Mediating the miR-423-5p/SOX4 Signaling. Int. J. Biol. Markers 36 (4), 10–20. doi:10.1177/17246008211043128
Wen, Y., Wang, Y., Xing, Z., Liu, Z., and Hou, Z. (2018). Microarray Expression Profile and Analysis of Circular RNA Regulatory Network in Malignant Pleural Effusion. Cell. Cycle 17 (24), 2819–2832. doi:10.1080/15384101.2018.1558860
Wesselhoeft, R. A., Kowalski, P. S., and Anderson, D. G. (2018). Engineering Circular RNA for Potent and Stable Translation in Eukaryotic Cells. Nat. Commun. 9 (1), 2629. doi:10.1038/s41467-018-05096-6
Woodrum, D. T., and Gauger, P. G. (2005). Role of131I in the Treatment of Well Differentiated Thyroid Cancer. J. Surg. Oncol. 89 (3), 114–121. doi:10.1002/jso.20185
Wu, F., Li, F., Lin, X., Xu, F., Cui, R.-R., Zhong, J.-Y., et al. (2019). Exosomes Increased Angiogenesis in Papillary Thyroid Cancer Microenvironment. Endocr. Relat. Cancer 26 (5), 525–538. doi:10.1530/erc-19-0008
Wu, G., Zhou, W., Pan, X., Sun, Z., Sun, Y., Xu, H., et al. (2020a). RETRACTED: Circular RNA Profiling Reveals Exosomal Circ_0006156 as a Novel Biomarker in Papillary Thyroid Cancer. Mol. Ther. - Nucleic Acids 19, 1134–1144. doi:10.1016/j.omtn.2019.12.025
Wu, P., Fang, X., Liu, Y., Tang, Y., Wang, W., Li, X., et al. (2021). N6-methyladenosine Modification of circCUX1 Confers Radioresistance of Hypopharyngeal Squamous Cell Carcinoma through Caspase1 Pathway. Cell. Death Dis. 12 (4), 298. doi:10.1038/s41419-021-03558-2
Wu, X., Bian, B., Lin, Z., Wu, C., Sun, Y., Pan, Y., et al. (2022). Identification of Exosomal mRNA, lncRNA and circRNA Signatures in an Osteoarthritis Synovial Fluid-Exosomal Study. Exp. Cell. Res. 410 (1), 112881. doi:10.1016/j.yexcr.2021.112881
Xia, F., Chen, Y., Jiang, B., Bai, N., and Li, X. (2020). Hsa_circ_0011385 Accelerates the Progression of Thyroid Cancer by Targeting miR-361-3p. Cancer Cell. Int. 20, 49. doi:10.1186/s12935-020-1120-7
Xia, S., Feng, J., Lei, L., Hu, J., Xia, L., Wang, J., et al. (2017). Comprehensive Characterization of Tissue-specific Circular RNAs in the Human and Mouse Genomes. Brief. Bioinform 18 (6), bbw081–992. doi:10.1093/bib/bbw081
Xiao, Q., Zhong, J., Tang, X., and Luo, J. (2021). iCDA-CMG: Identifying circRNA-Disease Associations by Federating Multi-Similarity Fusion and Collective Matrix Completion. Mol. Genet. Genomics 296 (1), 223–233. doi:10.1007/s00438-020-01741-2
Xie, Z., Gao, Y., Ho, C., Li, L., Jin, C., Wang, X., et al. (2022). Exosome-delivered CD44v6/C1QBP Complex Drives Pancreatic Cancer Liver Metastasis by Promoting Fibrotic Liver Microenvironment. Gut 71 (3), 568–579. doi:10.1136/gutjnl-2020-323014
Xing, M., Alzahrani, A. S., Carson, K. A., Viola, D., Elisei, R., Bendlova, B., et al. (2013). Association between BRAF V600E Mutation and Mortality in Patients with Papillary Thyroid Cancer. Jama 309 (14), 1493–1501. doi:10.1001/jama.2013.3190
Xiong, H., Yu, H., Jia, G., Yu, J., Su, Y., Zhang, J., et al. (2021a). circZFR Regulates Thyroid Cancer Progression by the miR ‐16/MAPK1 axis. Environ. Toxicol. 36 (11), 2236–2244. doi:10.1002/tox.23337
Xiong, H., Yu, J., Jia, G., Su, Y., Zhang, J., Xu, Q., et al. (2021b). Emerging Roles of circUBAP2 Targeting miR-370-3p in Proliferation, Apoptosis, and Invasion of Papillary Thyroid Cancer Cells. Hum. Cell. 34 (6), 1866–1877. doi:10.1007/s13577-021-00585-1
Xu, Y., Leng, K., Yao, Y., Kang, P., Liao, G., Han, Y., et al. (2021). A Circular RNA, Cholangiocarcinoma‐Associated Circular RNA 1, Contributes to Cholangiocarcinoma Progression, Induces Angiogenesis, and Disrupts Vascular Endothelial Barriers. Hepatology 73 (4), 1419–1435. doi:10.1002/hep.31493
Xue, C., Cheng, Y., Wu, J., Ke, K., Miao, C., Chen, E., et al. (2020). Circular RNA CircPRMT5 Accelerates Proliferation and Invasion of Papillary Thyroid Cancer through Regulation of miR-30c/E2F3 Axis. Cmar Vol. 12, 3285–3291. doi:10.2147/cmar.S249237
Yang, C., Wei, Y., Yu, L., and Xiao, Y. (2019). Identification of Altered Circular RNA Expression in Serum Exosomes from Patients with Papillary Thyroid Carcinoma by High-Throughput Sequencing. Med. Sci. Monit. 25, 2785–2791. doi:10.12659/msm.915658
Yang, D., Jin, Y., Cheng, S., and Yang, Y. (2020a). The Interaction between Circular RNA Hsa_circ_0000285 and miR-599 in Thyroid Cancer. Eur. Rev. Med. Pharmacol. Sci. 24 (13), 4882–4889. doi:10.26355/eurrev_202007_2187010.26355/eurrev_202005_21177
Yang, J., Cao, X.-H., Luan, K.-F., and Huang, Y.-D. (2021). Circular RNA FNDC3B Protects Oral Squamous Cell Carcinoma Cells from Ferroptosis and Contributes to the Malignant Progression by Regulating miR-520d-5p/SLC7A11 Axis. Front. Oncol. 11, 672724. doi:10.3389/fonc.2021.672724
Yang, L., Han, B., Zhang, Z., Wang, S., Bai, Y., Zhang, Y., et al. (2020b). Extracellular Vesicle-Mediated Delivery of Circular RNA SCMH1 Promotes Functional Recovery in Rodent and Nonhuman Primate Ischemic Stroke Models. Circulation 142 (6), 556–574. doi:10.1161/circulationaha.120.045765
Yang, W., Bai, C., Zhang, L., Li, Z., Tian, Y., Yang, Z., et al. (2020c). Correlation between Serum circRNA and Thyroid Micropapillary Carcinoma with Cervical Lymph Node Metastasis. Med. Baltim. 99 (47), e23255. doi:10.1097/md.0000000000023255
Yang, Y.-S., Jia, X.-Z., Lu, Q.-Y., Cai, S.-L., Huang, X.-T., Yang, S.-H., et al. (2022). Exosomal DEK Removes Chemoradiotherapy Resistance by Triggering Quiescence Exit of Breast Cancer Stem Cells. Oncogene 41 (18), 2624–2637. doi:10.1038/s41388-022-02278-x
Yang, Y., Ding, L., Li, Y., and Xuan, C. (2020d). Hsa_circ_0039411 Promotes Tumorigenesis and Progression of Papillary Thyroid Cancer by miR‐1179/ABCA9 and miR‐1205/MTA1 Signaling Pathways. J. Cell. Physiology 235 (2), 1321–1329. doi:10.1002/jcp.29048
Yang, Z., Shi, J., Xie, J., Wang, Y., Sun, J., Liu, T., et al. (2020e). Large-scale Generation of Functional mRNA-Encapsulating Exosomes via Cellular Nanoporation. Nat. Biomed. Eng. 4 (1), 69–83. doi:10.1038/s41551-019-0485-1
Yao, Y., Chen, X., Yang, H., Chen, W., Qian, Y., Yan, Z., et al. (2019). Hsa_circ_0058124 Promotes Papillary Thyroid Cancer Tumorigenesis and Invasiveness through the NOTCH3/GATAD2A axis. J. Exp. Clin. Cancer Res. 38 (1), 318. doi:10.1186/s13046-019-1321-x
Ye, M., Hou, H., Shen, M., Dong, S., and Zhang, T. (2020). Circular RNA circFOXM1 Plays a Role in Papillary Thyroid Carcinoma by Sponging miR-1179 and Regulating HMGB1 Expression. Mol. Ther. - Nucleic Acids 19, 741–750. doi:10.1016/j.omtn.2019.12.014
Yoo, S.-K., Song, Y. S., Lee, E. K., Hwang, J., Kim, H. H., Jung, G., et al. (2019). Integrative Analysis of Genomic and Transcriptomic Characteristics Associated with Progression of Aggressive Thyroid Cancer. Nat. Commun. 10 (1), 2764. doi:10.1038/s41467-019-10680-5
Yu, W., Ma, B., Zhao, W., Liu, J., Yu, H., Tian, Z., et al. (2020). The Combination of circRNA-UMAD1 and Galectin-3 in Peripheral Circulation Is a Co-biomarker for Predicting Lymph Node Metastasis of Thyroid Carcinoma. Am. J. Transl. Res. 12 (9), 5399–5415.
Zang, J., Lu, D., and Xu, A. (2020). The Interaction of circRNAs and RNA Binding Proteins: An Important Part of circRNA Maintenance and Function. J. Neurosci. Res. 98 (1), 87–97. doi:10.1002/jnr.24356
Zeng, L., Yuan, S., Zhou, P., Gong, J., Kong, X., and Wu, M. (2021). Circular RNA Pvt1 Oncogene (CircPVT1) Promotes the Progression of Papillary Thyroid Carcinoma by Activating the Wnt/β-Catenin Signaling Pathway and Modulating the Ratio of microRNA-195 (miR-195) to Vascular Endothelial Growth Factor A (VEGFA) Expression. Bioengineered 12 (2), 11795–11810. doi:10.1080/21655979.2021.2008639
Zhang, D., Tang, J., Kong, D., Cui, Q., Wang, K., Gong, Y., et al. (2018a). Impact of Gender and Age on the Prognosis of Differentiated Thyroid Carcinoma: a Retrospective Analysis Based on SEER. Horm. Canc 9 (5), 361–370. doi:10.1007/s12672-018-0340-y
Zhang, D., Tao, L., Xu, N., Lu, X., Wang, J., He, G., et al. (2022). CircRNA circTIAM1 Promotes Papillary Thyroid Cancer Progression through the miR-646/HNRNPA1 Signaling Pathway. Cell. Death Discov. 8 (1), 21. doi:10.1038/s41420-021-00798-1
Zhang, H., Ma, X. P., Li, X., and Deng, F. S. (2019). Circular RNA Circ_0067934 Exhaustion Expedites Cell Apoptosis and Represses Cell Proliferation, Migration and Invasion in Thyroid Cancer via Sponging miR-1304 and Regulating CXCR1 Expression. Eur. Rev. Med. Pharmacol. Sci. 23 (24), 10851–10866. doi:10.26355/eurrev_201912_19789
Zhang, H., Xiao, X., Wei, W., Huang, C., Wang, M., Wang, L., et al. (2021a). CircLIFR Synergizes with MSH2 to Attenuate Chemoresistance via MutSα/ATM-P73 axis in Bladder Cancer. Mol. Cancer 20 (1), 70. doi:10.1186/s12943-021-01360-4
Zhang, Q., Wu, L., Liu, S.-Z., Chen, Q.-J., Zeng, L.-P., Chen, X.-Z., et al. (2021b). Hsa_circ_0023990 Promotes Tumor Growth and Glycolysis in Dedifferentiated TC via Targeting miR-485-5p/FOXM1 Axis. Endocrinology 162 (12). doi:10.1210/endocr/bqab172
Zhang, S., Wang, Q., Li, D., Huang, B., Hou, X., Wang, D., et al. (2020a). Oncolytic Vaccinia Virus-Mediated Antitumor Effect and Cell Proliferation Were Promoted in PTC by Regulating circRNA_103598/miR-23a-3p/IL-6 Axis. Cmar Vol. 12, 10389–10396. doi:10.2147/cmar.S273072
Zhang, W., Liu, T., Li, T., and Zhao, X. (2021c). Hsa_circRNA_102002 Facilitates Metastasis of Papillary Thyroid Cancer through Regulating miR-488-3p/HAS2 axis. Cancer Gene Ther. 28 (3-4), 279–293. doi:10.1038/s41417-020-00218-z
Zhang, W., Zhang, H., and Zhao, X. (2020b). circ_0005273 Promotes Thyroid Carcinoma Progression by SOX2 Expression. Endocr. Relat. Cancer 27 (1), 11–21. doi:10.1530/erc-19-0381
Zhang, X.-O., Dong, R., Zhang, Y., Zhang, J.-L., Luo, Z., Zhang, J., et al. (2016). Diverse Alternative Back-Splicing and Alternative Splicing Landscape of Circular RNAs. Genome Res. 26 (9), 1277–1287. doi:10.1101/gr.202895.115
Zhang, Y., Zhang, G., Liu, Y., Chen, R., Zhao, D., McAlister, V., et al. (2018b). GDF15 Regulates Malat-1 Circular RNA and Inactivates NFκB Signaling Leading to Immune Tolerogenic DCs for Preventing Alloimmune Rejection in Heart Transplantation. Front. Immunol. 9, 2407. doi:10.3389/fimmu.2018.02407
Zhang, Y., Zhang, X.-O., Chen, T., Xiang, J.-F., Yin, Q.-F., Xing, Y.-H., et al. (2013). Circular Intronic Long Noncoding RNAs. Mol. Cell. 51 (6), 792–806. doi:10.1016/j.molcel.2013.08.017
Zhang, Z., Wang, W., Su, Z., Zhang, J., and Cao, H. (2021d). Circ_0011058 Facilitates Proliferation, Angiogenesis and Radioresistance in Papillary Thyroid Cancer Cells by Positively Regulating YAP1 via Acting as miR-335-5p Sponge. Cell. Signal. 88, 110155. doi:10.1016/j.cellsig.2021.110155
Zheng, F. B., Chen, D., Ding, Y. Y., Wang, S. R., Shi, D. D., and Zhu, Z. P. (2020). Circular RNA Circ_0103552 Promotes the Invasion and Migration of Thyroid Carcinoma Cells by Sponging miR-127. Eur. Rev. Med. Pharmacol. Sci. 24 (5), 2572–2578. doi:10.26355/eurrev_202003_20526
Zheng, H., Fu, Q., Ma, K., Shi, S., and Fu, Y. (2021a). Circ_0079558 Promotes Papillary Thyroid Cancer Progression by Binding to miR-26b-5p to Activate MET/AKT Signaling. Endocr. J. 68 (11), 1247–1266. doi:10.1507/endocrj.EJ20-0498
Zheng, L.-L., Li, J.-H., Wu, J., Sun, W.-J., Liu, S., Wang, Z.-L., et al. (2016). deepBase v2.0: Identification, Expression, Evolution and Function of Small RNAs, LncRNAs and Circular RNAs from Deep-Sequencing Data. Nucleic Acids Res. 44 (D1), D196–D202. doi:10.1093/nar/gkv1273
Zheng, X., Rui, S., Wang, X.-F., Zou, X.-H., Gong, Y.-P., and Li, Z.-H. (2021b). circPVT1 Regulates Medullary Thyroid Cancer Growth and Metastasis by Targeting miR-455-5p to Activate CXCL12/CXCR4 Signaling. J. Exp. Clin. Cancer Res. 40 (1), 157. doi:10.1186/s13046-021-01964-0
Zhou, G. K., Zhang, G. Y., Yuan, Z. N., Pei, R., and Liu, D. M. (2018). Has_circ_0008274 Promotes Cell Proliferation and Invasion Involving AMPK/mTOR Signaling Pathway in Papillary Thyroid Carcinoma. Eur. Rev. Med. Pharmacol. Sci. 22 (24), 8772–8780. doi:10.26355/eurrev_201812_16644
Zhou, H., He, X., He, Y., Ou, C., and Cao, P. (2021a). Exosomal circRNAs: Emerging Players in Tumor Metastasis. Front. Cell. Dev. Biol. 9, 786224. doi:10.3389/fcell.2021.786224
Zhou, Y., Yu, Z., Wang, X., Chen, W., Liu, Y., Zhang, Y., et al. (2021b). Exosomal circRNAs Contribute to Intestinal Development via the VEGF Signalling Pathway in Human Term and Preterm Colostrum. Aging 13 (8), 11218–11233. doi:10.18632/aging.202806
Zhu, J., Wang, Y., Yang, C., Feng, Z., Huang, Y., Liu, P., et al. (2021). circ-PSD3 Promoted Proliferation and Invasion of Papillary Thyroid Cancer Cells via Regulating the miR-7-5p/METTL7B axis. J. Recept. Signal Transduct. 42, 251–260. doi:10.1080/10799893.2021.1910706
Glossary
TC Thyroid cancer
circRNAs Circular RNAs
RBPs RNA binding proteins
PTC Papillary thyroid cancer
DTCs Differentiated thyroid cancers
EcircRNAs Exonic circular RNAs
ciRNAs Intronic circular RNAs
EIciRNAs Exon-intron circular RNAs
MecciRNAs Mitochondria-encoded circular RNAs
TricRNAs Pre-tRNA circular RNAs
tRNA transfer RNA
RNA Pol II RNA polymerase II
ICSs Intronic complementary sequences
QKI Quaking
m6A N6-methyladenosine
ceRNA Competitive endogenous RNA
miRNA microRNA
AGO2 Argonaute 2
YTHDF2 YTH domain-containing family protein 2
UPF1 Upstream frameshift 1
RNase H1 Ribonuclease H1
ncRNAs Noncoding RNAs
LncRNA Long noncoding RNA
DECs Differentially expressed circRNAs
FC Fold change
LNM Lymphnode metastasis
EMT Epithelial-mesenchymal transformation
AhR Aryl hydrocarbon receptor
PDK Pyruvate dehydrogenase kinase
AUC Area under the receiver operative characteristic curve
ROC Receiver operative characteristic curve
OS Overall survival
DFS Disease free survival
CI Confidence interval
RR Relative risk
HR Hazard ratio
PDX Patient-derived xenograf
BSJ Back spliced junction
PKR Protein kinase R.
Keywords: thyroid cancer, circular RNAs, dysregulation, function, mechanism, perspective
Citation: Yao X and Zhang Q (2022) Function and Clinical Significance of Circular RNAs in Thyroid Cancer. Front. Mol. Biosci. 9:925389. doi: 10.3389/fmolb.2022.925389
Received: 21 April 2022; Accepted: 22 June 2022;
Published: 22 July 2022.
Edited by:
Liang Chen, University of Science and Technology of China, ChinaReviewed by:
Ricardo Cortez Cardoso Penha, International Agency For Research On Cancer (IARC), FranceBingbing Ren, Zhejiang University, China
Copyright © 2022 Yao and Zhang. This is an open-access article distributed under the terms of the Creative Commons Attribution License (CC BY). The use, distribution or reproduction in other forums is permitted, provided the original author(s) and the copyright owner(s) are credited and that the original publication in this journal is cited, in accordance with accepted academic practice. No use, distribution or reproduction is permitted which does not comply with these terms.
*Correspondence: Qiu Zhang, zhangqiu@ahmu.edu.cn