Altered Energy Mobilization Within the Littoral Food Web in New Habitat Created by Climate-Induced Changes in Lake Water Level
- 1State Key Laboratory of Freshwater Ecology and Biotechnology of China, Institute of Hydrobiology, Chinese Academy of Sciences, Wuhan, China
- 2College of Advanced Agricultural Sciences, University of Chinese Academy of Sciences, Beijing, China
- 3Research Monitoring and Evaluation Center of Qinghai National Park, Xining, China
Littoral zones in oligotrophic lakes play an essential role in supporting animal consumers and in the exchange of matter between the water body and the terrestrial sources, but are easily altered by changes in water level. We studied Qinghai Lake, a deep oligotrophic lake in northwest China, where lake water level has increased rapidly in recent years, altering the character of the littoral zones. We sampled common organisms and used stable carbon and nitrogen isotope analyses to compare how contributions of different sources (allochthonous and autochthonous) to the diets of consumers differed between sand (original habitat, OH) and submerged grassland (new habitat, NH) substrate habitat conditions. Our results showed that allochthonous resources (i.e., terrestrial detritus) constituted the largest diet proportion of consumers in OH due to poor nutrient conditions, while consumers in NH utilized more autochthonous resources (i.e., Cladophora and phytoplankton). We also found that terrestrial nutrient subsidies from soil and decomposed grass led to increased biomasses of Cladophora, phytoplankton, zooplankton and zoobenthos in NH compared to those in OH, accounting for autochthonous replacement of part of the allochthonous resources in NH. Therefore, hydrological conditions may alter the trophic interactions within littoral food webs, contributing to a more complex and interconnected food web. Overall, our results suggest that the littoral food webs of Qinghai Lake are vulnerable to changes in hydrological conditions, which may be enhanced by climate change.
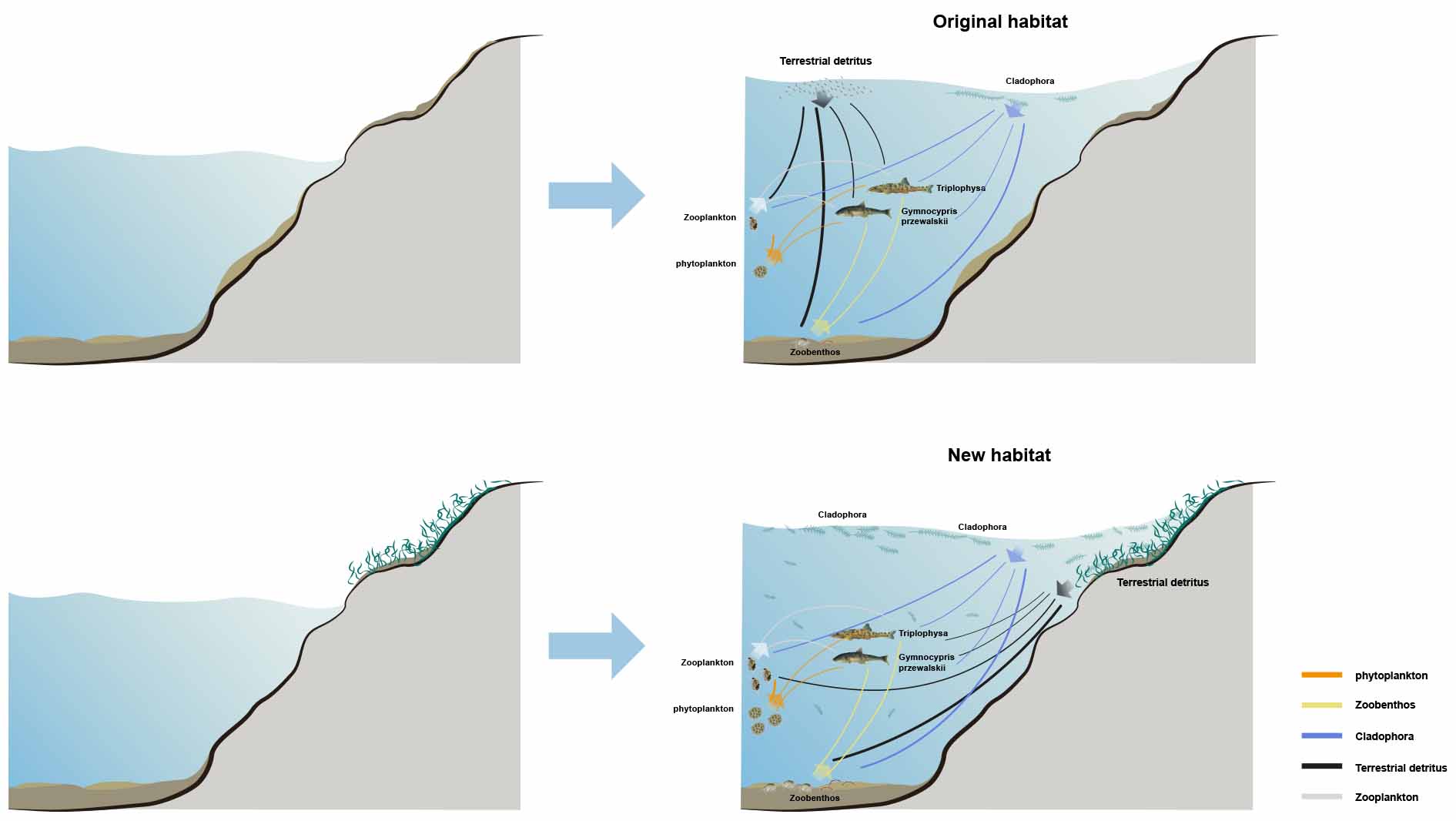
Graphical Abstract. The dynamic changes in littoral food web interaction strengths and energy pathways under the two different habitat status.
Introduction
Lakes provide a range of services for human beings, and are also a key component of global biogeochemical, ecological and hydrological processes (Fan et al., 2021). Lakes are also sensitive to changes in climate (Tao et al., 2020; Woolway et al., 2020), which can lead to significant changes in the water level, water storage and inundation area of lakes (Tao et al., 2020). For instance, the amount of water storage on the Tibetan Plateau has increased, and most lakes in the region are increasing in size in response to these changes (Huang et al., 2011; Zhang et al., 2017). Such changes in water level may be expected to create new habitats in the littoral zones and to modify existing habitats, and hence may trigger responses in littoral food webs by affecting the growth of littoral phytoplankton and the quantity and quality of terrestrial sources exported to lakes (Wiegner et al., 2009).
Changes in hydrology and climate collectively contribute to transformations of the landscape, which lead to alterations in the catchment vegetation. Delivery of nutrients and organic materials across distinct habitat boundaries constitute ecosystem subsidy, and cross-ecosystem nutrient subsidies can have strong effects on lake systems by changing nutrient limitation and increasing autochthonous resources (Brahney et al., 2015; Slemmons et al., 2015). Therefore, cross-ecosystem nutrient subsidies caused by factors such as climate change may have far-reaching consequences on freshwater ecosystems. Indeed, there is evidence that allochthonous terrestrial subsidies increase secondary production in lakes (Pace et al., 2007), especially in lakes with lower productivity, where most of the food resources used by consumers can be underpinned by terrestrial subsidies (Karlsson et al., 2012). The water flow from the inundation area brings a large amount of dissolved and particulate organic matter of terrestrial origin, stimulates primary productivity, and the quality and quantity of food can increase in nutrient-limited systems ultimately supporting higher trophic levels (Klug, 2002; Finstad et al., 2014).
Food webs are fundamental to ecosystem functioning because their structure controls fluxes of energy and supports key processes such as productivity (Albouy et al., 2014). Any change in energy flow can affect the wider lake ecosystem function. For example, changes in primary production associated with variation in energy flows have a large bottom-up effect from primary consumers to the fish community, which may lead to large-scale re-structuring of the whole food web (Xu et al., 2014). Nakano et al. (1999) suggested that the predation pressure of fish shifted rapidly from terrestrial to aquatic arthropods when terrestrial arthropod inputs to the stream, which can provide energy subsidies, were reduced and the resulting impact may cascade eventually affect primary producers through the food web.
The extent to which littoral communities can be subsidized by increased energy flows, and the impact of such allochthonous inputs on the food webs through cascading trophic interaction are still poorly understood. Indeed, the dynamic features of the food web, such as the trophic interactions and energy flux, are still one of the least understood subjects in aquatic ecosystems (Xu et al., 2014; Mao et al., 2021). Qinghai Lake, in the northeast of the Tibetan Plateau, provides a good site for empirical studies to address this gap in understanding. The water level of Qinghai Lake has experienced substantial changes over the past 50 years with the water level having risen by nearly 3 m between 2004 and 2018 (Zhang et al., 2014; Dong et al., 2019), so there is a considerable flux of energy and nutrients from the surrounding terrestrial landscape to the lake’s littoral habitats. However, some littoral zones in the lake are not fundamentally affected by the rise of water level where the area that has been inundated has basically the same substrata as the original littoral. Hence, because the terrestrial subsidies vary spatially due to the spatial heterogeneity of physical and geographical elements, this lake is an ideal ecosystem for examining alterations in energy mobilization within littoral food webs.
We measured stable C and N isotopes of food web components to identify which kind of resources were the main contributors to the diets of local consumers. We hypothesized that: (i) the lack of the availability of autochthonous resources in original habitat and the increase in the availability of allochthonous resources have resulted in a greater demand for terrestrial subsidies by consumers than in new habitat; and (ii) compared with original habitat, the biomass of phytoplankton and zooplankton in new habitat will be larger because of the existence of more terrestrial nutrient subsidies, which will ultimately support fishes. To test these hypotheses, we established the food web structures for old and new habitats, characterized the contributions of allochthonous and autochthonous resources to consumers respectively, and focused on the dynamic changes in littoral food web interaction strengths and energy pathways under the two different habitat states.
Materials and Methods
Study Site and Sample Collection
Qinghai Lake (36°32′-37°15′N, 99°36′-100°16′E) is located in the northeast part of the Tibet Plateau. with the water level at 3,196 m asl. It is the largest saline lake in China with a surface area of approximately 4,472 km2 (measured in 2018) (Fan et al., 2021), and is surrounded by alpine meadows. Due to the rapid increase of water level, a large inundation area has appeared around the lake and two types of habitats have formed. One habitat substratum is sand, which is similar to that in the main lake body and hence can be regarded as an outward expansion of the original littoral habitat of Qinghai Lake (original habitat, OH); the other habitat substratum is submerged grassland (new habitat, NH). The bottom topography of both habitats is flat (depth range 1.0–1.2 m).
Cladophora filaments were sparsely scattered in OH, whereas NH with submerged grassland supports large amounts of Cladophora. No growth of macrophytes was observed in either habitat. We collected food web samples for this study from the two different habitats in July 2020 by dividing each habitat into 10 equal sites and sampling them so as to ensure that the collected samples accurately represent the ecological characteristics of the habitat (Supplementary Figure 1). In addition, fishes, benthic invertebrates, plankton, Cladophora and terrestrial detritus were sampled for stable isotope analyses to determine changes in consumer diets and food web structure in the different habitats. Water samples were collected by mixing the surface (0.6 m below the surface), middle and bottom water column (0.6 m above the bottom) in 2 L pre-cleaned polyethylene bottles, and total phosphorus (TP) and nitrogen (TN) concentrations were measured using methods developed by Kotlash and Chessman (1998). Cladophora samples were collected by plant grab (0.5 m × 0.4 m) at each site and we measured their fresh biomass after removing sediment and water.
Zooplankton and phytoplankton samples for biomass calculation were collected by sieving 20 L surface water through a 112-μm mesh size plankton net and sieving 1 L surface water through a 64-μm mesh size plankton net respectively, and all the material were preserved with 4% Lugol’s iodine solution. The Lugol samples were placed under laboratory conditions, and after more than 48 h of standing and precipitation, concentrated into 30 ml for identification and counting under the microscope, and assume that 1 mm3 of volume is equivalent to 1 mg of wet weights biomass. Wet weights of the zooplankton were calculated from the length-weight regressions of Huang et al. (1999); and wet weights of the phytoplankton were calculated from the cell numbers and size measurements (Huang et al., 1999).
Shed leaves from the most abundant grassland species including Achnatherum splendens and Orinus kokonorica surrounding the shoreline in OH were collected as potential terrestrial detritus, as these are more easily carried by the wind or washed into the lake than fresh leaves. Owing to the emergence of inundation areas there was a deep layer of terrestrial detritus on the bottom of NH, so decomposing grass leaves were also collected, and impurities and zoobenthos were removed. Cladophora was collected by hand and washed with distilled water and a soft toothbrush to remove silt and other impurities.
Zooplankton samples for stable isotope analysis a were collected using a 112-μm mesh size plankton net and then particulate contaminants were removed through visual inspection (Mack et al., 2012). The filtered water through a 64-μm mesh size plankton net again to collects phytoplankton samples for stable isotope analysis and then predatory zooplankton and particulate contaminants with large individuals were removed through visual inspection (Li et al., 2020).
Benthic invertebrates were obtained from both habitats using an Ekman grab (1/16 m2) and washed with a hand net (mesh size 1 mm), including Chironomus salinarius and Gammarus suifunensis which were dominant in these areas (Wang et al., 2021), and these were the only species chosen for subsequent stable isotope analysis. For all invertebrate species, the masses of single sample were not sufficient for analyses, so it was necessary to prepare homogenous samples of pooled individuals.
Zooplankton and benthic invertebrates were washed and kept in distilled water for 12 h to enable gut evacuation (Wang et al., 2021), in order to remove any bias caused by the gut contents themselves. Using distilled water for gut evacuation experiments might cause osmotic stress and thus changes in respiration for both zooplankton and benthic invertebrates. However, the exposure time (12 h) is likely not long enough to cause any significant changes in isotopic signatures. Zooplankton samples processed by gut evacuation and phytoplankton samples for stable isotope analysis were then filtered onto a pre-combusted (450°C, 4 h) Whatman GF/F filter. The GF/F filter of phytoplankton samples used for stable isotope analysis were examined under microscopic to remove protozoa and rotifers to reduce the impact of mixed samples.
Fish, including Gymnocypris przewalskii and Triplophysa, were captured using gill nets and traps from two random transects from littoral zones in each habitat, nets and traps were in place for approximately 24 h. The weight of fish caught of G. przewalskii and Triplophysa in OH were 4.44 and 0.31 kg respectively, and 4.79 and 0.6 kg in NH. We collected white dorsal muscle samples from the larger fish individuals (>100 mm) and homogenized separately for stable isotope analysis. This reduces possible bias caused by foraging shifts by fish individuals in their early life cycles (Xu et al., 2014).
We also determine water turbidity in two habitats by Hach 2100Q portable turbidimetry.
Stable Isotope Analysis and Modeling
All samples for stable isotope analysis were stored frozen until analysis. Terrestrial detritus was treated with 1 M hydrochloric acid to eliminate possible carbonate contamination and then rinsed in distilled water (Wang et al., 2021). All samples were dried to constant weight at 60°C, then ground into a homogeneous fine powder with a mortar and pestle and finally packed separately in tin capsules. The stable C and N isotope ratio values were generated after analysis of samples at the Institute of Hydrobiology, Chinese Academy of Sciences, using a Delta Plus (Finnigan, Bremen, Germany) continuous-flow isotope ratio mass spectrometer coupled to a Carlo Erba NA2500 elemental analyzer (Carlo Erba Reagenti, Milan, Italy). Stable isotope ratios were expressed in δ notation as parts per thousand (‰) deviation from the international standards according to the equation: δX = [(Rsample/Rstandard) - 1] × 1,000, where X is 15N or 13C and R is the corresponding ratio 15N/14N or 13C/12C. δ is the measure of heavy to light isotope in the sample, whereby higher δ values denote a greater proportion of the heavy isotope. The standard reference material for carbon was Vienna Pee Dee Belemnite and for nitrogen was atmospheric nitrogen (N2). The reference material for δ13C was carbonate (IAEA–USGS24) and for δ15N was ammonium sulfate (IAEA-USGS26), supplied by the US Geological Survey (Denver, Colombia) and certified by the International aAomic Energy Agency (Vienna, Austria). Urea (δ15N = -1.53‰, δ13C = -49.44‰) was used as the daily internal working standard. Twenty percent of the samples were run as replicates, and the average standard errors of replicate measurements for δ13C and δ15N were both less than 0.2‰.
The trophic enrichment factors (TEF), caused by isotope fractionation during the process of digestion and metabolism, of Δδ13C = 1.70 ± 0.40‰ and Δδ15N = 2.80 ± 0.30‰ as proposed by Arcagni et al. (2013) were used to correct stable isotope values of food sources. Potential food sources for consumers were chosen based on published diet information (Drenner et al., 1996; Jacobsen et al., 2017). We chose terrestrial detritus, phytoplankton and Cladophora as food sources for zooplankton, zoobenthos, Triplophysa and G. przewalskii; zooplankton and zoobenthos were also included as food sources for Triplophysa and G. przewalskii.
Statistics
The trophic relationships among the species were compared visually through stable isotope biplots of consumers and potential food sources. Before analysis, the normality and heteroscedasticity of relevant data were evaluated by Shapiro–Wilk test and Bartlett test (Mao et al., 2021). Student’s t-tests were also used to test the differences in the biomass of phytoplankton, zooplankton, zoobenthos, and Cladophora, the concentration of total nitrogen, total phosphorus, stable isotope values of species and turbidity between the two habitats. All statistical analyses were set at α = 0.05 statistical significance levels.
We used an expanded Bayesian isotope mixing model (BIMM) to estimate dietary proportions of every consumers in the food web to obtain a quantitative food web implemented in the “IsoWeb” package (Kadoya et al., 2012). This model required information for stable isotope values of all consumers and resources, and a topological web to generate hypothetical dietary proportions for the food web of each habitat. This approach takes into account variation in trophic enrichment factors among different consumer-resource links to achieve more accurate estimates (Kadoya et al., 2012). BIMM fitting was implemented using the “R2WinBugs” package in R version 4.0.3 for Windows (Team, 2020); the accuracy of the model was determined by comparing the difference between the isotopic values of samples and the predicted values of the model. In order to ensure the convergence of the three chains, the parameters in the Markov Chain Monte Carlo were specified as chain length 10,000 burn-in 5,000 and thin by 10. Through the output of posterior distributions, we could then calculate the mean, standard deviation, and Bayesian credible intervals for the contribution of potential prey sources to consumers.
We estimated the mean contribution of sources to the diet of each consumer, and then we compared the distributions of values for the different habitats to examine differences in source utilization and energy mobilization. When calculating the difference between two states, effect sizes based on standardized differences between the means are typically used (Fritz et al., 2012). Cohen (2013) introduced a measure parameter D, which was calculated according to the following equation:
where D is effect sizes, M1, M2 and S1, S2 are the means and the standard deviation for the two groups respectively (Fritz et al., 2012). Three levels of size of effect to be detected were set: small (0–0.2), medium (0.21–0.5), and large (0.51 and above); a larger effect size indicates a greater difference, and a bigger impact of the variable causing a difference (Cohen, 1962).
Results
There was no significant difference in water depth between OH and NH (Supplementary Table 1) and both habitats were crystal clear, with no difference in transparency evident to the naked eye. However, both the water chemistry and biomasses of major resources in NH differed significantly from those in OH. TP in NH was 0.14 mg L–1 higher than in OH (t-test, p < 0.01), but TN differed little (Figure 1A and Supplementary Table 1). The rise of water level resulted in the appearance of submerged grassland e in large areas in NH, with an accompanying higher phytoplankton biomass (t-test, p < 0.01), zooplankton biomass (t-test, p = 0.021), zoobenthos biomass and Cladophora biomass (t-test, p < 0.01), with Cladophora especially increasing from 3.62 g m–2 in OH to 41.33 g m–2 in NH (Figures 1B–D and Supplementary Table 1).
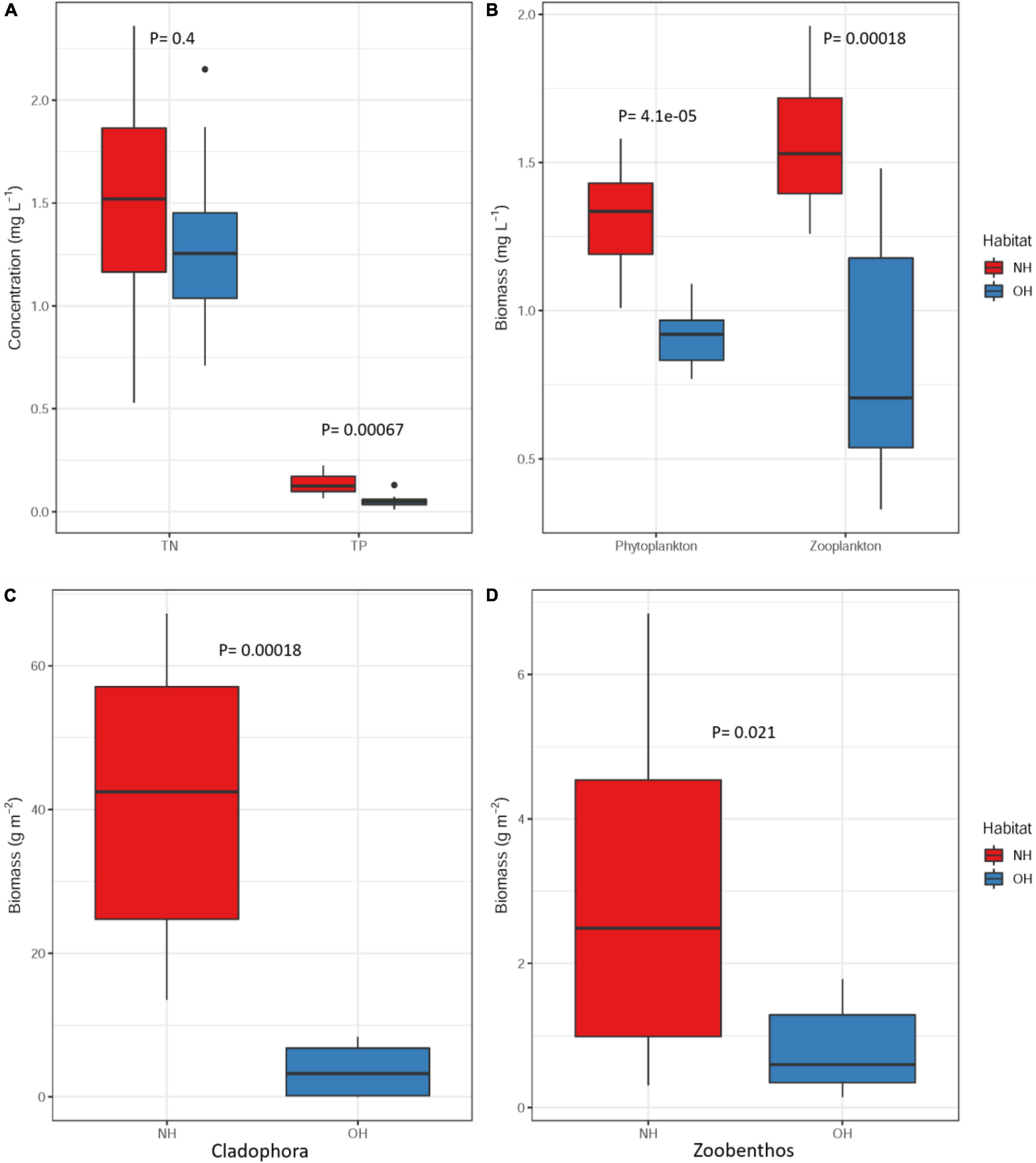
Figure 1. Changes in total phosphorus and total nitrogen (A), phytoplankton and zooplankton biomass (B), Cladophora biomass (C), and zoobenthos biomass (D) in Qinghai Lake between original (blue) and new (red) habitats. P-values are marked above the box plots.
Supplementary Tables 2, 3 summarize the isotope values of all samples collected from OH and NH. Comparison of the isotope space biplots of each habitat (Figure 2) indicates that almost all taxa from NH tend to have higher δ15N and δ13C values than their counterparts in OH. Within the basal sources, phytoplankton and Cladophora from NH had significantly higher δ15N and δ13C values than in OH (t-test p < 0.05), but no differences were observed in δ15N and δ13C values of terrestrial detritus between the two habitats (Supplementary Table 4). In both habitats, invertebrates and fishes in NH showed significantly higher δ15N and δ13C values than in OH (Supplementary Table 4). Triplophysa and G. przewalskii from within the same habitat had similar δ15N values (11.37 ± 0.34‰ in Triplophysa and 11.18 ± 0.42‰ in G. przewalskii), suggesting closely similar feeding habits.
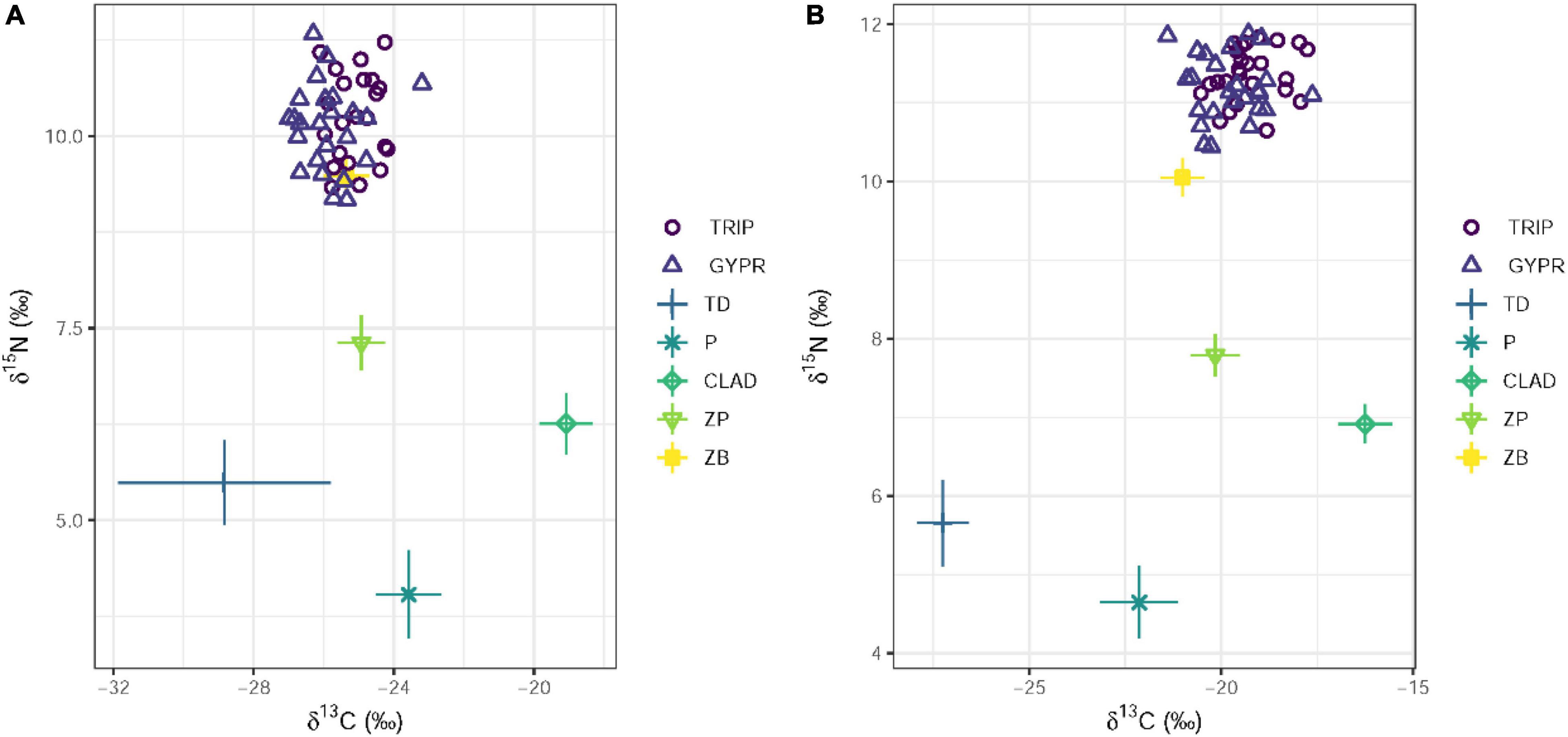
Figure 2. Raw (not including trophic discrimination factors) stable carbon and nitrogen isotope values (mean ± SD) of basal food sources and consumers in original (A) and new (B) habitats. TRIP, Triplophysa; GYPR, Gymnocypris przewalskii; ZP, zooplankton; ZB, zoobenthos; P, phytoplankton; CLAD, Cladophora; TD, terrestrial detritus.
BIMM was used to estimate the relative contribution of the various resources to consumers. The isotope values from OH and NH food webs were treated as observed values and compared with predicted values from the BIMM estimates and the observed points broadly lie in the predicted value intervals, showing that the model can make reasonably accurate estimates (Figure 3). According to the results of the BIMM, trophic interactions of food web components in the two habitats are different. In the OH, terrestrial detritus showed the highest contribution to the diet of zooplankton (42%), zoobenthos (65%) and G. przewalskii (26%), and the contribution to Triplophysa (22%) also accounts for a considerable part compared with other resources, whereas Cladophora and phytoplankton contributed the least to G. przewalskii (14%, 14%) and Triplophysa (15%, 15%) (Table 1). Interestingly, in the NH, the dependence of all consumers on terrestrial detritus decreased in varying degrees, replaced by an increase in Cladophora and phytoplankton assimilation, which was most obvious in zooplankton and zoobenthos. Zooplankton in NH heavily reduced dependence on terrestrial detritus (down from 42 to 27%), and increased their dependence on Cladophora (up from 21 to 26%) and phytoplankton (up from 37 to 47%) at the same time (Table 1). Zoobenthos in NH also reduced dependence on terrestrial detritus (down from 65 to 58%), and increased their dependence on Cladophora (up from 35 to 42%). Zooplankton and zoobenthos were important to fishes; however, the support by zooplankton for Triplophysa tended to be similar between habitats, whereas zoobenthos contributed more to G. przewalskii (up from 25 to 28%) and Triplophysa (up from 25 to 31%) in NH (Table 1).
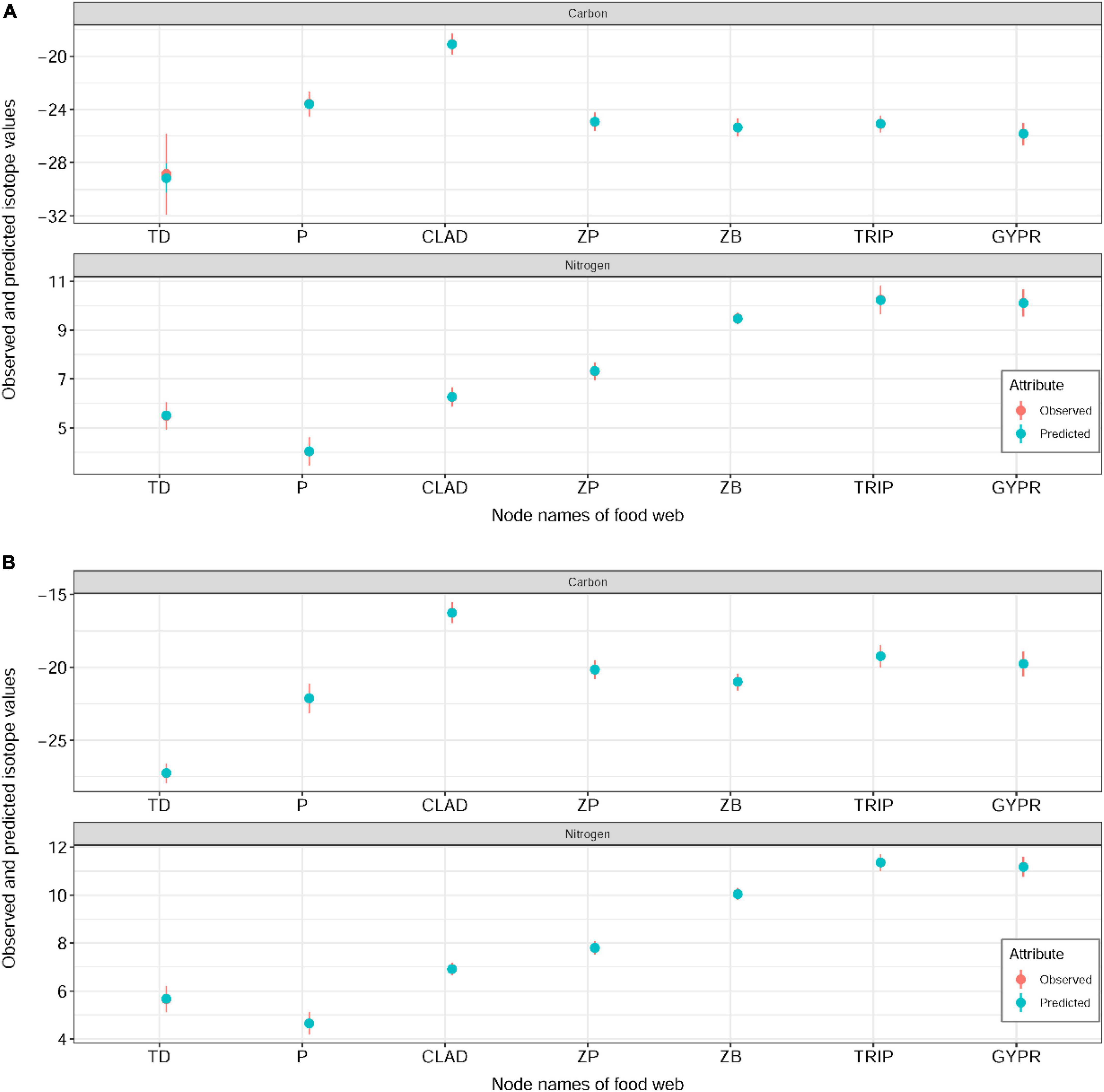
Figure 3. Comparison of model predictions (blue) and observations (red) in original (A) and new (B) habitats. The solid points show the medians of observed and predicted values, whereas error bars represent 95% confidence intervals for each source.
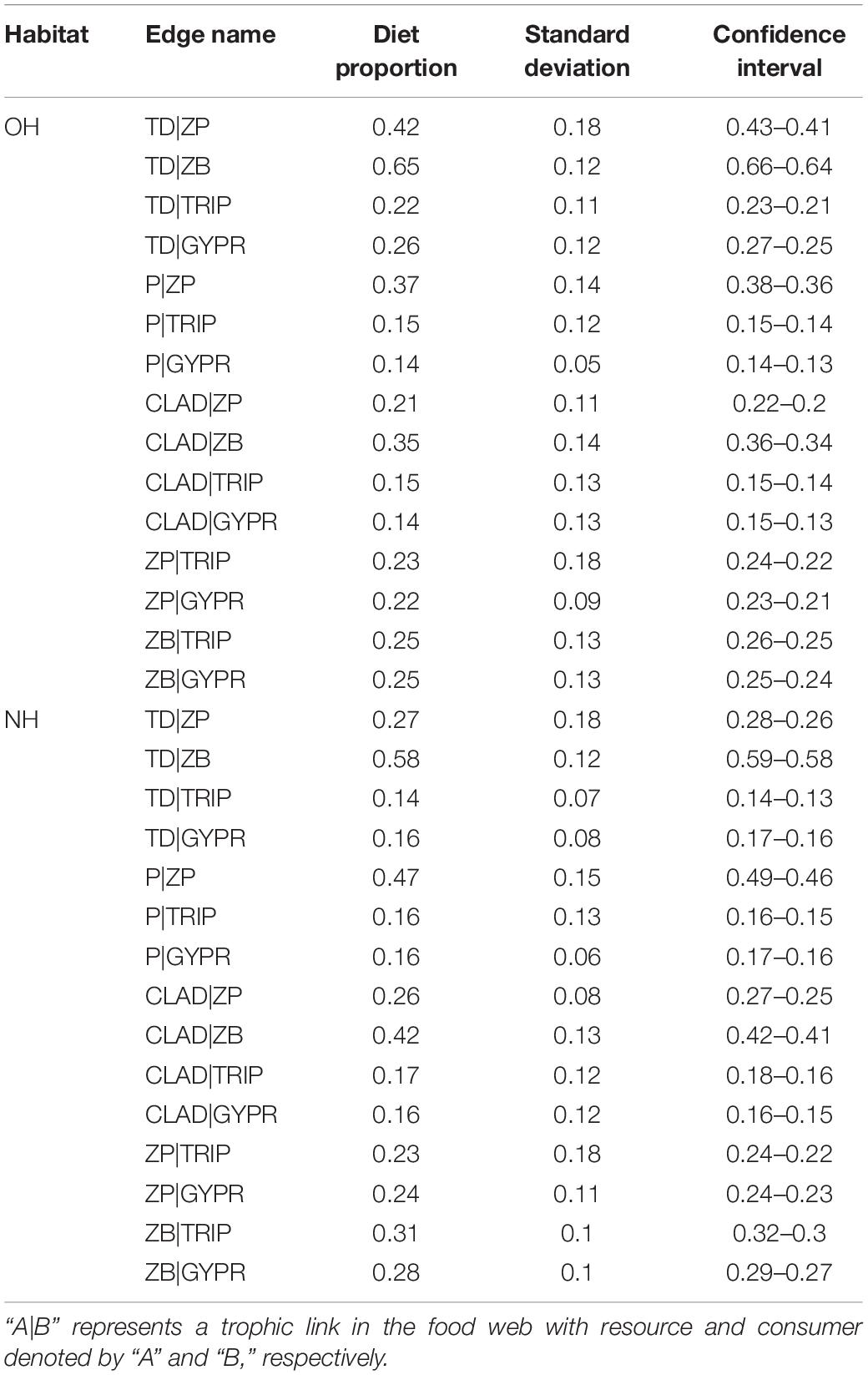
Table 1. Bayesian isotope mixing model estimates of diet proportions for consumers in original (OH) and new (NH) habitats.
Using effect sizes to measure the changes in the interaction strength of trophic links between the two habitats, a conceptualized diagram was constructed for visualization (Figures 4, 5). It can be seen that, except for the trophic links related to terrestrial detritus, the interaction strength of the other links was strong in NH, especially the links between Cladophora and zooplankton (0.53, medium effect), Cladophora and zoobenthos (0.51, medium effect), phytoplankton and zooplankton (0.69, medium effect), and zoobenthos and Triplophysa (0.52, medium effect). However, the difference in interaction strength of trophic links related to terrestrial detritus between two habitats were almost the largest, as seen from the links between terrestrial detritus and zooplankton (-0.83, large effect), zoobenthos (-0.58, medium effect), Triplophysa (-0.87, large effect) and G. przewalskii (-0.98, large effect) (Figures 4, 5). This indicates that the interaction strength of food webs with similar trophic structures shows different intensity in the two habitats. For most consumers in OH, autochthonous resources were the most important carbon source, while allochthonous resources contributed most to consumers in NH (Table 1).
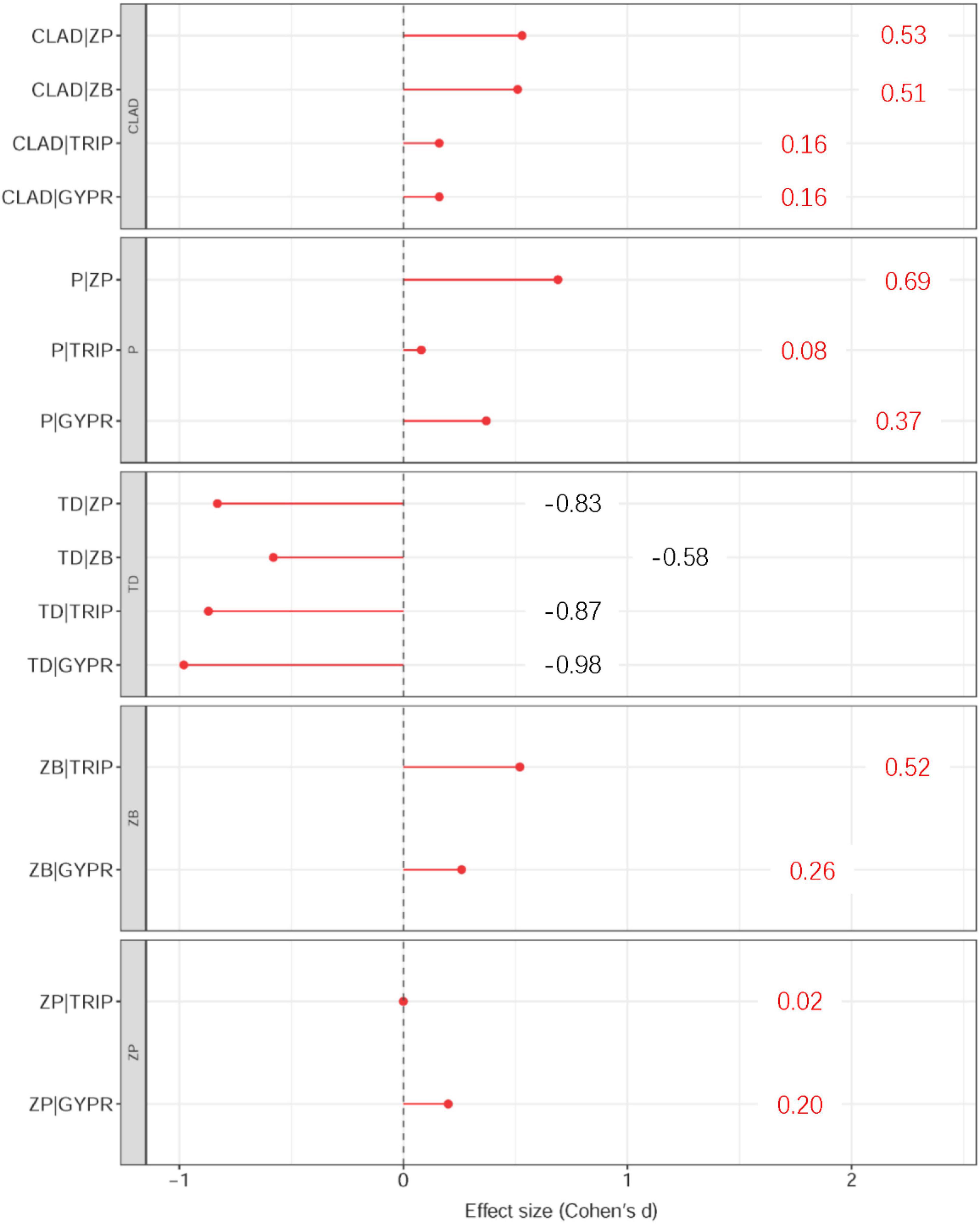
Figure 4. Mean effect sizes of the difference in interaction strength of the same trophic links between the two habitats. Positive effects sizes indicate that the link is stronger in the new habitat whereas negative effect sizes indicate that the link is stronger in the original habitat.
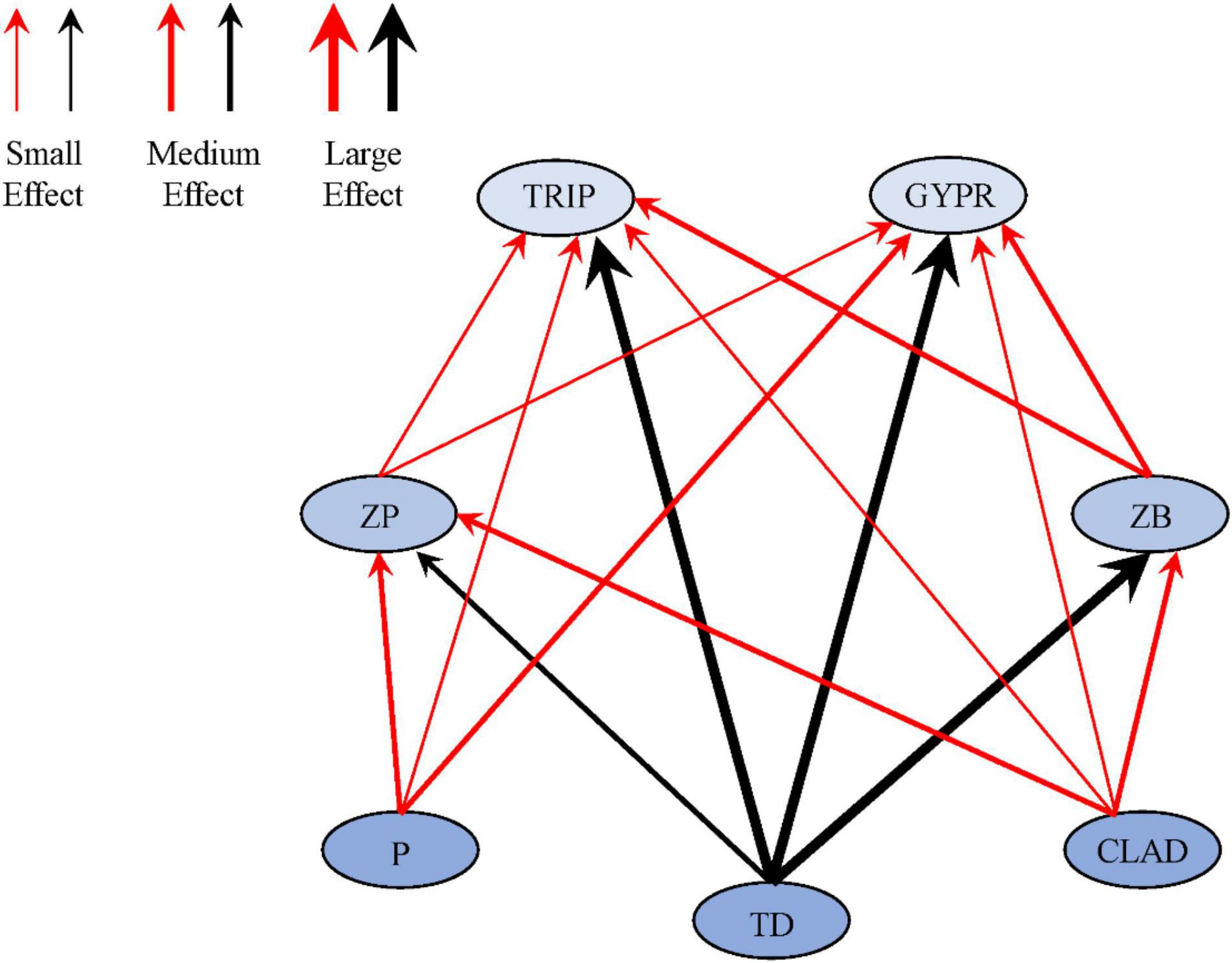
Figure 5. Schematic diagram of the strengths of trophic links investigated in this study. The width of the arrows between resources and consumers reflects estimated effect sizes: small (0–0.2), medium (0.21–0.5), and large (0.51 and above). Positive effects sizes and negative effects sizes are represented in red and black, respectively.
Discussion
Changes in Habitats
Ongoing climate change can affect lake water level fluctuations through increasing abnormally wet years, glacier meltwater and other factors (Tao et al., 2020; Fan et al., 2021), which in turn affects the ecological processes and patterns of lake littoral zones. However, our understanding of the mechanisms underlying these affects is still limited. The resulting inundation areas will form new aquatic habitats and feeding grounds, and the nutrient load released from soil particles and organic matter will also increase (Steinman et al., 2012), providing nutrients directly or indirectly for the local habitat. Indeed, due to nutrient inputs, the concentrations of total phosphorus, phytoplankton and Cladophora biomass in NH were significantly increased compare with OH, whereas total nitrogen did not change (Figures 1A–C). Even so, like many other alpine lakes, these two littoral habitats still belong to the low nutrient category (Supplementary Table 1), and we did not find any sign of regime shift from clear to turbid water caused by nutrient released to water columns (Jeppesen et al., 1998), Hence, even though the biomass of phytoplankton did increase, t Cladophora in NH probably quickly incorporates most additional nutrients near shoreline development. The unnatural Cladophora blooms in this clear lake can be explained by the availability of nutrients and substrata. Increased algal biomass at NH strongly suggests that these sites receive increased nutrients. Compared with the sand substratum in OH, we conclude that submerged grassland in NH, which has higher phosphorus content, provided enough nutrients for growth of Cladophora (Figure 1A). Moreover, the relatively high transparency and the low water depth (<1.3 m) of the two habitats allows enough light penetration to the lake bottom to support growth of Cladophora. Previous studies have shown that phosphate is the limiting factor for the growth of Cladophora (Ren et al., 2019), and the Cladophora biomass of Qinghai Lake was positively correlated with total phosphate concentrations, whereas no obvious correlation was observed with total nitrogen (Zhu et al., 2020), which is also consistent with our findings.
In addition to nutrients, submerged grassland substratum in NH provided additional attachment points for the growth of Cladophora enabling them to expand into areas that were previously difficult to colonize (Francoeur et al., 2017).
Carbon Sources for Consumers
Inundation areas may increase the exchange of matter and energy between the terrestrial and aquatic environments in the littoral zones of lakes and thus change the basal food sources supporting the food web (Leira and Cantonati, 2008). In Qinghai Lake the contributions of allochthonous and autochthonous carbon to consumers were different in different habitats. BIMM estimates revealed that the most important carbon source for zooplankton, zoobenthos and fishes in OH was terrestrial detritus, i.e., allochthonous carbon, whereas in NH, it was autochthonous carbon and especially Cladophora (Table 1). In fact, we also found that δ13C values of zoobenthos and fishes showed an obvious trend away from values of terrestrial detritus toward values of Cladophora (Supplementary Table 1), which means that compared with OH, consumers in NH potentially had assimilated considerable amount of carbon derived from Cladophora. This change in the contributions of allochthonous and autochthonous carbon sources to consumers can be explained by resource availability. Food webs in oligotrophic lakes commonly rely strongly on terrestrially produced organic matter because of their poor nutritional condition (Jonsson et al., 2001). In OH the low production of autochthonous carbon sources such as phytoplankton and Cladophora (Figures 1B,C) is insufficient to maintain the biomass of consumer species; instead, we believe that the large inputs of terrestrial detritus from nearshore grassland provides a sufficient food source to subsidize the growth of consumers in OH. With the increase of submerged grassland area and amount in NH, these subsidies have not become more important, which we consider may be caused by two reasons. Firstly, as mentioned above, nutrient input from soil particles and decomposed grass supports the growth of autochthonous carbon sources in NH, which replace a considerable part of allochthonous carbon sources in the food web. Secondly, algae are considered higher-quality food for consumers than terrestrial detritus, because of their higher content of polyunsaturated fatty acids required to meet physiological requirements (Lau et al., 2009; Guo et al., 2016). More Cladophora and phytoplankton in NH increased availability of high-quality food sources, which consumers preferred. Thus, autochthonous carbon resources were ingested more than allochthonous carbon resources in NH. In addition, we emphasize that allochthonous resources subsidize rather than entirely replacing autochthonous resources, and habitat characteristics also partly determine the strength of this subsidy, which has proven to be closely related to climate change (Bartley et al., 2019).
Shift in Energetic Mobilization of Food Webs
Cross-ecosystem nutrient subsidies tend to bring nutrients from areas with higher productivity into areas with lower productivity, and these inputs can alter habitat stability, which causes corresponding changes in interaction strength and food web structure (Huxel and McCann, 1998; Atwood et al., 2012). For example, omnivorous fish in the Pantanal wetland have been observed to respond to flooding-induced changes in energy pathways and nutrients by ingesting more plant food (Wantzen et al., 2002), which indicates that omnivorous fish can reduce this effect through establishing direct or indirect links with a variety of energy subsidies (Polis and Strong, 1996). Therefore, we believe that the increased contribution of autochthonous resources to higher consumers in NH after the allochthonous nutrient inputs will help stabilize the food web. Following the increased availability of autochthonous resources in NH, the need for consumers to use terrestrial subsidies to maintain secondary production will be alleviated (Brett et al., 2017).
Zooplankton and zoobenthos are recognized as critical links between primary production and higher consumers, and are considered high-quality aquatic prey which can change the food base of fishes by delivering more carbon sources from primary producers to higher consumers (Vander Zanden and Vadeboncoeur, 2002; Mao et al., 2021). The diet proportions of fish may depend on the availability of food resources in the littoral zone, and the increase in allochthonous nutrients in the lake basin may have a positive effect. Supporting this view is the fact that support by phytoplankton, Cladophora, zooplankton, and zoobenthos resources for G. przewalskii and Triplophysa all showed positive effects, although to varying degrees (Figure 5). With the increased biomass of phytoplankton and Cladophora, more autochthonous resources were consumed by the zooplankton and zoobenthos subsequently preyed on by fishes (Figures 1B,C). Accordingly, the contribution of phytoplankton and Cladophora to zooplankton and zoobenthos also showed a positive effect (Figure 5). These shifts in trophic interactions and energy flux were reflected by the variation in δ15N values of related species; for instance, significantly higher δ15N values were observed for the fishes in NH (Supplementary Table 4). Consumers could gain more effective energy subsidies from zooplankton and zoobenthos, which were animal items and had a higher δ15N value than other resources, which may influence fish prey selection (Mao et al., 2021). Therefore, increased allochthonous nutrient support for autochthonous resources, and then transfer along the food chain could be an important part of the reason for maintaining the stability of the food web in NH.
At the same time, we also found that the contribution of terrestrial detritus decreased when the contribution of autochthonous resource increased, suggesting that the use of terrestrial subsidies by aquatic consumers depends in part on the stock biomass of aquatic prey (Kraus et al., 2016). Unlike the situation in OH where species consumed more terrestrial detritus to cope with a low resource availability, the availability of autochthonous resource in NH was much higher, and could replace part of the terrestrial subsidies. Nutritional asymmetry between aquatic and terrestrial resources in consumers is also an important factor (Twining et al., 2019).
Conclusion
In this study, we demonstrated that the water level fluctuations in Qinghai Lake caused by climate change have a far-reaching impact on its littoral habitat. Allochthonous inputs supported consumers in OH directly due to poor nutrient conditions and supported consumers in NH indirectly by improving the relative availability of autochthonous resources. Our results emphasize that lake and littoral ecosystems should be regarded as an integrated management unit, because the integrity of one is strongly associated with the other (Larsen et al., 2016). Moreover, terrestrial subsidies should attract greater concern when formulating lake ecosystem management strategy.
Data Availability Statement
The original contributions presented in this study are included in the article/Supplementary Material, further inquiries can be directed to the corresponding author/s.
Ethics Statement
This animal study was reviewed and approved by the Research Monitoring and Evaluation Center of Qinghai National Park.
Author Contributions
JX and KW conceived the study. KW, KZ, XX, HZu, HA, and KM conducted field and laboratory measurements. JX and KW analyzed the data. All authors drafted and revised the manuscript, contributed to the article, and approved the submitted version.
Funding
This research was supported by the National Key R&D Program of China (Grant No. 2018YFD0900904) and the Water Pollution Control and Management Project of China (Grant No. 2018ZX07208005). JX acknowledges the support received from the International Cooperation Project of the Chinese Academy of Sciences (Grant No. 152342KYSB20190025) and the National Natural Science Foundations of China (Grant No. 31872687).
Conflict of Interest
The authors declare that the research was conducted in the absence of any commercial or financial relationships that could be construed as a potential conflict of interest.
Publisher’s Note
All claims expressed in this article are solely those of the authors and do not necessarily represent those of their affiliated organizations, or those of the publisher, the editors and the reviewers. Any product that may be evaluated in this article, or claim that may be made by its manufacturer, is not guaranteed or endorsed by the publisher.
Acknowledgments
KW acknowledges designer Jin Wang from Bauhaus University for her efforts in graphical abstract.
Supplementary Material
The Supplementary Material for this article can be found online at: https://www.frontiersin.org/articles/10.3389/fevo.2022.886372/full#supplementary-material
References
Albouy, C., Velez, L., Coll, M., Colloca, F., Le Loc’h, F., Mouillot, D., et al. (2014). From projected species distribution to food-web structure under climate change. Glob. Chang. Biol. 20, 730–741. doi: 10.1111/gcb.12467
Arcagni, M., Campbell, L. M., Arribere, M. A., Kyser, K., Klassen, K., Casaux, R., et al. (2013). Food web structure in a double-basin ultra-oligotrophic lake in Northwest Patagonia, Argentina, using carbon and nitrogen stable isotopes. Limnologica 43, 131–142. doi: 10.1016/j.limno.2012.08.009
Atwood, T. B., Wiegner, T. N., and MacKenzie, R. A. (2012). Effects of hydrological forcing on the structure of a tropical estuarine food web. Oikos 121, 277–289. doi: 10.1111/j.1600-0706.2011.19132.x
Bartley, T. J., McCann, K. S., Bieg, C., Cazelles, K., Granados, M., Guzzo, M. M., et al. (2019). Food web rewiring in a changing world. Nat. Ecol. Evol. 3, 345–354. doi: 10.1038/s41559-018-0772-3
Brahney, J., Mahowald, N., Ward, D. S., Ballantyne, A. P., and Neff, J. C. (2015). Is atmospheric phosphorus pollution altering global alpine Lake stoichiometry? Glob. Biogeochem. Cycles 29, 1369–1383. doi: 10.1002/2015GB005137
Brett, M. T., Bunn, S. E., Chandra, S., Galloway, A. W. E., Guo, F., Kainz, M. J., et al. (2017). How important are terrestrial organic carbon inputs for secondary production in freshwater ecosystems? Freshw. Biol. 62, 833–853. doi: 10.1111/fwb.12909
Cohen, J. (1962). The statistical power of abnormal-social psychological research: a review. J. Abnorm. Soc. Psychol. 65, 145–153. doi: 10.1037/h0045186
Cohen, J. (2013). Statistical Power analysis for the Behavioral Sciences. Cambridge, MA: Academic Press. doi: 10.4324/9780203771587
Dong, H., Song, Y., and Zhang, M. (2019). Hydrological trend of Qinghai Lake over the last 60 years: driven by climate variations or human activities? J. Water Clim. Change 10, 524–534. doi: 10.2166/wcc.2018.033
Drenner, R. W., Smith, J. D., and Threlkeld, S. T. (1996). Lake trophic state and the limnological effects of omnivorous fish. Hydrobiologia 319, 213–223. doi: 10.1007/BF00013734
Fan, C., Song, C., Li, W., Liu, K., Cheng, J., Fu, C., et al. (2021). What drives the rapid water-level recovery of the largest lake (Qinghai Lake) of China over the past half century? J. Hydrol. 593:125921. doi: 10.1016/j.jhydrol.2020.125921
Finstad, A. G., Helland, I. P., Ugedal, O., Hesthagen, T., and Hessen, D. O. (2014). Unimodal response of fish yield to dissolved organic carbon. Ecol. Lett. 17, 36–43. doi: 10.1111/ele.12201
Francoeur, S. N., Winslow, K. A. P., Miller, D., and Peacor, S. D. (2017). Mussel-derived stimulation of benthic filamentous algae: the importance of nutrients and spatial scale. J. Great Lakes Res. 43, 69–79. doi: 10.1016/j.jglr.2016.10.013
Fritz, C. O., Morris, P. E., and Richler, J. (2012). Effect size estimates: current use, calculations, and interpretation. J. Exp. Psychol. Gen. 141, 2–18. doi: 10.1037/a0024338
Guo, F., Kainz, M. J., Sheldon, F., and Bunn, S. E. (2016). The importance of high-quality algal food sources in stream food webs - current status and future perspectives. Freshw. Biol. 61, 815–831. doi: 10.1111/fwb.12755
Huang, L., Liu, J., Shao, Q., and Liu, R. (2011). Changing inland lakes responding to climate warming in Northeastern Tibetan Plateau. Clim. Change 109, 479–502. doi: 10.1007/s10584-011-0032-x
Huang, X., Chen, W., and Cai, Q. (1999). Survey, observation and analysis of lake ecology. Standard methods for observation analysis in Chinese Ecosystem Research Network, Series V.
Huxel, G. R., and McCann, K. (1998). Food web stability: the influence of trophic flows across habitats. Am. Nat. 152, 460–469. doi: 10.1086/286182
Jacobsen, D., Laursen, S. K., Hamerlik, L., Moltesen, K., Michelsen, A., and Christoffersen, K. S. (2017). Fish on the roof of the world: densities, habitats and trophic position of stone loaches (Triplophysa) in Tibetan streams. Mar. Freshw. Res. 68, 53–64. doi: 10.1071/MF15225
Jeppesen, E., Sondergaard, M., Jensen, J. P., Mortensen, E., Hansen, A. M., and Jorgensen, T. (1998). Cascading trophic interactions from fish to bacteria and nutrients after reduced sewage loading: an 18-year study of a shallow hypertrophic lake. Ecosystems 1, 250–267. doi: 10.1007/s100219900020
Jonsson, A., Meili, M., Bergstrom, A. K., and Jansson, M. (2001). Whole-lake mineralization of allochthonous and autochthonous organic carbon in a large Humic lake (Ortrasket, N. Sweden). Limnol. Oceanogr. 46, 1691–1700. doi: 10.4319/lo.2001.46.7.1691
Kadoya, T., Osada, Y., and Takimoto, G. (2012). IsoWeb: a Bayesian isotope mixing model for diet analysis of the whole food web. PLoS One 7:e41057. doi: 10.1371/journal.pone.0041057
Karlsson, J., Berggren, M., Ask, J., Bystrom, P., Jonsson, A., Laudon, H., et al. (2012). Terrestrial organic matter support of lake food webs: evidence from lake metabolism and stable hydrogen isotopes of consumers. Limnol. Oceanogr. 57, 1042–1048. doi: 10.4319/lo.2012.57.4.1042
Klug, J. L. (2002). Positive and negative effects of allochthonous dissolved organic matter and inorganic nutrients on phytoplankton growth. Can. J. Fish. Aquat. Sci. 59, 85–95. doi: 10.1139/f01-194
Kotlash, A. R., and Chessman, B. C. (1998). Effects of water sample preservation and storage on nitrogen and phosphorus determinations: implications for the use of automated sampling equipment. Water Res. 32, 3731–3737. doi: 10.1016/S0043-1354(98)00145-6
Kraus, J. M., Pomeranz, J. F., Todd, A. S., Walters, D. M., Schmidt, T. S., and Wanty, R. B. (2016). Aquatic pollution increases use of terrestrial prey subsidies by stream fish. J. Appl. Ecol. 53, 44–53. doi: 10.1111/1365-2664.12543
Larsen, S., Muehlbauer, J. D., and Marti, E. (2016). Resource subsidies between stream and terrestrial ecosystems under global change. Glob. Change Biol. 22, 2489–2504. doi: 10.1111/gcb.13182
Lau, D. C. P., Leung, K. M. Y., and Dudgeon, D. (2009). Are autochthonous foods more important than allochthonous resources to benthic consumers in tropical headwater streams? J. N. Am. Benthol. Soc. 28, 426–439. doi: 10.1899/07-079.1
Leira, M., and Cantonati, M. (2008). Effects of water-level fluctuations on lakes: an annotated bibliography. Hydrobiologia 613, 171–184. doi: 10.1007/s10750-008-9465-2
Li, Y. R., Meng, J., Zhang, C., Ji, S. P., Kong, Q., Wang, R. Q., et al. (2020). Bottom-up and top-down effects on phytoplankton communities in two freshwater lakes. PLoS One 15:e0231357. doi: 10.1371/journal.pone.0231357
Mack, H. R., Conroy, J. D., Blocksom, K. A., Stein, R. A., and Ludsin, S. A. (2012). A comparative analysis of zooplankton field collection and sample enumeration methods. Limnol. Oceanogr. Methods 10, 41–53. doi: 10.4319/lom.2012.10.41
Mao, Z., Gu, X., Cao, Y., Luo, J., Zeng, Q., Chen, H., et al. (2021). Pelagic energy flow supports the food web of a shallow lake following a dramatic regime shift driven by water level changes. Sci. Total Environ. 756:143642. doi: 10.1016/j.scitotenv.2020.143642
Nakano, S., Miyasaka, H., and Kuhara, N. (1999). Terrestrial-aquatic linkages: riparian arthropod inputs alter trophic cascades in a stream food web. Ecology 80, 2435–2441. doi: 10.1890/0012-9658(1999)080[2435:TALRAI]2.0.CO;2
Pace, M. L., Carpenter, S. R., Cole, J. J., Coloso, J. J., Kitchell, J. F., Hodgson, J. R., et al. (2007). Does terrestrial organic carbon subsidize the planktonic food web in a clear-water lake? Limnol. Oceanogr. 52, 2177–2189. doi: 10.4319/lo.2007.52.5.2177
Polis, G. A., and Strong, D. R. (1996). Food web complexity and community dynamics. Am. Nat. 147, 813–846. doi: 10.1086/285880
Ren, Z., Niu, D. C., Ma, P. P., Wang, Y., Fu, H., and Elser, J. J. (2019). Cascading influences of grassland degradation on nutrient limitation in a high mountain lake and its inflow streams. Ecology 100:e02755. doi: 10.1002/ecy.2755
Slemmons, K. E. H., Saros, J. E., Stone, J. R., McGowan, S., Hess, C. T., and Cahl, D. (2015). Effects of glacier meltwater on the algal sedimentary record of an alpine lake in the central US Rocky Mountains throughout the late Holocene. J. Paleolimnol. 53, 385–399. doi: 10.1007/s10933-015-9829-3
Steinman, A. D., Ogdahl, M. E., Weinert, M., Thompson, K., Cooper, M. J., and Uzarski, D. G. (2012). Water level fluctuation and sediment-water nutrient exchange in Great Lakes coastal wetlands. J. Great Lakes Res. 38, 766–775. doi: 10.1016/j.jglr.2012.09.020
Tao, S., Fang, J., Ma, S., Cai, Q., Xiong, X., Tian, D., et al. (2020). Changes in China’s lakes: climate and human impacts. Natl. Sci. Rev. 7, 132–140. doi: 10.1093/nsr/nwz103
Team, R. D. C. (2020). R: A Language and Environment for Statistical Computing. Vienna, Austria: R Foundation for Statistical Computing.
Twining, C. W., Brenna, J. T., Lawrence, P., Winkler, D. W., Flecker, A. S., and Hairston, N. G. Jr. (2019). Aquatic and terrestrial resources are not nutritionally reciprocal for consumers. Funct. Ecol. 33, 2042–2052. doi: 10.1111/1365-2435.13401
Vander Zanden, M. J., and Vadeboncoeur, Y. (2002). Fishes as integrators of benthic and pelagic food webs in lakes. Ecology 83, 2152–2161. doi: 10.2307/3072047
Wang, K., Sha, Y. C., Xu, J., Zhang, T. L., Hu, W., and Zhu, Z. Y. (2021). Do sympatric transgenic and non-transgenic common carps partition the trophic niche? A whole-lake manipulation study. Sci. Total Environ. 787:147516. doi: 10.1016/j.scitotenv.2021.147516
Wantzen, K. M., Machado, F. D., Voss, M., Boriss, H., and Junk, W. J. (2002). Seasonal isotopic shifts in fish of the Pantanal wetland, Brazil. Aquat. Sci. 64, 239–251. doi: 10.1007/PL00013196
Wiegner, T. N., Tubal, R. L., and MacKenzie, R. A. (2009). Bioavailability and export of dissolved organic matter from a tropical river during base- and stormflow conditions. Limnol. Oceanogr. 54, 1233–1242. doi: 10.4319/lo.2009.54.4.1233
Woolway, R. I., Kraemer, B. M., Lenters, J. D., Merchant, C. J., O’Reilly, C. M., and Sharma, S. (2020). Global lake responses to climate change. Nat. Rev. Earth Environ. 1, 388–403. doi: 10.1038/s43017-020-0067-5
Xu, J., Wen, Z., Ke, Z., Zhang, M., Zhang, M., Guo, N., et al. (2014). Contrasting energy pathways at the community level as a consequence of regime shifts. Oecologia 175, 231–241. doi: 10.1007/s00442-013-2878-2
Zhang, G., Xie, H., Yao, T., Li, H., and Duan, S. (2014). Quantitative water resources assessment of Qinghai Lake basin using Snowmelt Runoff Model (SRM). J. Hydrol. 519, 976–987. doi: 10.1016/j.jhydrol.2014.08.022
Zhang, G., Yao, T., Shum, C., Yi, S., Yang, K., Xie, H., et al. (2017). Lake volume and groundwater storage variations in Tibetan Plateau’s endorheic basin. Geophys. Res. Lett. 44, 5550–5560. doi: 10.1002/2017GL073773
Keywords: climate change, food webs, stable isotopes, terrestrial subsidies, Cladophora, Qinghai Lake
Citation: Wang K, Zhao K, Xiong X, Zhu H, Ao H, Ma K, Xie Z, Wu C, Wang H, Zhang H, Zhang P and Xu J (2022) Altered Energy Mobilization Within the Littoral Food Web in New Habitat Created by Climate-Induced Changes in Lake Water Level. Front. Ecol. Evol. 10:886372. doi: 10.3389/fevo.2022.886372
Received: 28 February 2022; Accepted: 24 May 2022;
Published: 13 June 2022.
Edited by:
Gabriele Stowasser, British Antarctic Survey (BAS), United KingdomReviewed by:
Herwig Stibor, Ludwig Maximilian University of Munich, GermanyChristian Henri Nozais, Université du Québec à Rimouski, Canada
Copyright © 2022 Wang, Zhao, Xiong, Zhu, Ao, Ma, Xie, Wu, Wang, Zhang, Zhang and Xu. This is an open-access article distributed under the terms of the Creative Commons Attribution License (CC BY). The use, distribution or reproduction in other forums is permitted, provided the original author(s) and the copyright owner(s) are credited and that the original publication in this journal is cited, in accordance with accepted academic practice. No use, distribution or reproduction is permitted which does not comply with these terms.
*Correspondence: Huan Wang, wanghuan@ihb.ac.cn; Huan Zhang, zhanghuan@ihb.ac.cn; Peiyu Zhang, zhangpeiyu@ihb.ac.cn; Jun Xu, xujun@ihb.ac.cn