The Influence of Environmental Hypoxia on Hemostasis—A Systematic Review
- 1General and Surgical Intensive Care Medicine, Department of Anesthesiology and Critical Care Medicine, Medical University Innsbruck, Innsbruck, Austria
- 2Department of Anesthesiology and Critical Care Medicine, Medical University Innsbruck, Innsbruck, Austria
- 3Institute for Sports Medicine, Alpine Medicine and Health Tourism, Private University for Health Sciences, Medical Informatics and Technology UMIT, Hall i.T. and Tirol Kliniken GmbH, University Hospital Innsbruck, Innsbruck, Austria
Humans have been ascending to high altitudes for centuries, with a growing number of professional- and leisure-related sojourns occurring in this millennium. A multitude of scientific reports on hemostatic disorders at high altitude suggest that hypoxia is an independent risk factor. However, no systematic analysis of the influence of environmental hypoxia on coagulation, fibrinolysis and platelet function has been performed. To fill this gap, we performed a systematic literature review, including only the data of healthy persons obtained during altitude exposure (<60 days). The results were stratified by the degree of hypoxia and sub-categorized into active and passive ascents and sojourns. Twenty-one studies including 501 participants were included in the final analysis. Since only one study provided relevant data, no conclusions regarding moderate altitudes (1,500–2,500 m) could be drawn. At high altitude (2,500–5,400 m), only small pathophysiological changes were seen, with a possible impact of increasing exercise loads. Elevated thrombin generation seems to be balanced by decreased platelet activation. Viscoelastic methods do not support increased thrombogenicity, with fibrinolysis being unaffected by high altitude. At extreme altitude (5,400–8,850 m), the limited data showed activation of coagulation in parallel with stimulation of fibrinolysis. Furthermore, multiple confounding variables at altitude, like training status, exercise load, fluid status and mental stress, prevent definitive conclusions being drawn on the impact of hypoxia on hemostasis. Thus, we cannot support the hypothesis that hypoxia triggers hypercoagulability and increases the risk of thromboembolic disorders, at least in healthy sojourners.
Introduction
In the past few decades, a growing number of lowlanders have been sojourning at high altitudes for professional and leisure activities. The popularity of guided trekking tours at remote high-altitude regions remains high. However, some trekking tourists may suffer from lifestyle-related diseases, such as hypertension or obesity. Exposure to high altitudes, and the associated hypobaric hypoxia, induce a variety of physiological adaptations, a pivotal being the upregulation of hypoxia inducible transcription factors (HIFs) (1); maladaptation can also occur (e.g., acute mountain sickness), manifested as clinically relevant pathophysiological changes of the hemostatic system (2). These “coagulopathies” at high altitude mostly present as venous thrombosis or/and venous thromboembolism (VTE; deep venous thrombosis, pulmonary thromboembolism, mesenteric vein thrombosis, and cerebral venous sinus thrombosis [CVST]) (3–5). Cases of coronary stent thrombosis have even been reported, and cerebrovascular stroke occurring at high altitude was discussed in association with increased thrombogenicity (6). In the literature, a multitude of articles point to high altitude itself as an independent factor for adverse changes in the hemostatic system (7). Despite well-defined risk factors for VTE, little is known about the effects of high altitude on VTE risk in the general population; at present limited evidence exists to suggest a causal relationship. Various case reports have described patients who suffered from detrimental coagulation disorders at high altitude (8). A relationship between high altitude and CVST has long been suspected, based on anecdotal evidence from past case reports (9, 10). One study highlighted potential reversible risk factors for CVST occurring at high altitude, including smoking and dehydration. The increasing incidence of CVST has been associated with hypobaric hypoxia and strenuous exercise undertaken at peak summits. Pulmonary arterial embolism (PAE) is reported to be associated with high-altitude pulmonary edema (HAPE); however, the evidence indicates that PAE is not the cause, but rather the consequence, of HAPE. For all of these clinical findings, additional factors (e.g., extreme cold, exhaustion, a prolonged period of immobility in a tent, polycythemia and dehydration) were suggested to be contributory. A further confounding factor might be long-distance travel via aircraft, train, bus or car to remote mountain regions; such travel has been associated with the development of VTE (“travel thrombosis”) due to various factors (e.g., sitting in a cramped space during travel). Analysis of all existing reports on thromboembolism occurring at high altitude indicates that the majority of the patients suffered from preexisting medical conditions, i.e., inherent coagulation defects such as protein C (PC) and protein S (PS) deficiency, antiphospholipid syndromes and factor V Leiden mutation (5, 11, 12). Moreover, in patients with polycythemia vera residing at high altitude an association between altitude and an increased risk of thrombotic complications, most likely due to chronic increased HIF activation has been reported (13).
Despite this multitude of case reports and publications (albeit including small numbers of patients), there is no clear consensus regarding the risk of acquired hemostatic disorders at high altitude. This knowledge is crucial, because a growing number of people with and without preexisting diseases are traveling to high-altitude areas. At present, thorough scientific analysis of the influence of environmental hypoxia on coagulation, fibrinolysis and platelet function is lacking. In order to fill this gap, this systematic review aims to determine the influence of high-altitude hypoxia, typically encountered during trekking or mountaineering, on the hemostatic system. Chronic hypoxic exposure like in high-altitude dwellers or patients with congenital augmentation of hypoxia sensing are outside of the focus of this review.
Methodology
Protocol
To address the research question, a systematic literature review was performed. The method for identifying and including studies was consistent with the recommendations of the Cochrane Collaboration, as well as the guidelines of the Preferred Reporting Items for Systematic Reviews and Meta-Analyses Statement for Reporting Systematic Reviews (PRISMA).
Study Selection and Eligibility Criteria
This systematic search included literature from PubMed and the Cochrane Library (central register of controlled trials), and considered studies published from 1950 up until the 1st of July, 2021. We performed a broad and comprehensive search for articles and selected abstracts, titles and full texts using the following strategy: MeSh terms, i.e., “hypoxia,” “hypoxemia,” “altitude,” and “high altitude” were combined with OR and additionally combined with AND with the search block of “coagulation” OR “hemostasis” OR “fibrinolysis” (again, including text words and respective MeSh terms). Additionally, articles identified via manual search of the reference lists of included articles, as well as articles from grey literature (personal contacts of the authors), relevant to the topic were included.
Inclusion and Exclusion Criteria
Studies were required to meet the following inclusion criteria: (1) minimum altitude of 1,500 m above sea level, where altitude was classified as moderate (1,500–2,000 m); high (2,500–5,400 m) or extreme (5400–8850 m); (2) active (exercise during ascent and/or a sojourn) and passive hypoxic exposure (hypoxic chamber, passive ascent); (3) study participants were males or females aged over 18 years; and (4) original full-text articles written in English.
The following exclusion criteria were applied: (1) studies using animals or cell models; (2) short-term hypoxic exposure <6 h; (3) long-term stays (>60 days) above 2,500 m, to exclude high-altitude natives/residents and prolonged job-related sojourns (e.g., working in a hut or for the military); (4) intermittent bouts of hypoxic exposure; (5) studies including subjects exclusively in a sitting position during hypoxic exposure, to exclude venous stasis (“travel thrombosis”) as a potential confounder; (6) patients with preexisting diseases possibly interfering with hemostasis; (7) studies on HAPE and high-altitude cerebral edema (HACE) patients [although studies with a clearly defined asymptomatic population (control group) were included for further analysis]; and 8) any form of medical treatment interfering with hemostasis.
Data Extraction
The online tool Covidence (Covidence systematic review software, Veritas Health Innovation, Melbourne, Australia. www.covidence.org) was used for the screening, selection and classification of all articles. A total of 3,572 studies were identified in the initial search. In total, 58 duplicates were removed. The titles of the remaining articles were screened independently for suitability by two researchers (BT and BW). Any disagreements were resolved by discussion with a third author (WS). After screening 3,514 articles by title, 3,446 studies were excluded; the abstracts of the remaining 68 studies were screened for suitability. Of those, 48 were of further interest and their full texts were thus retrieved. One additional study was handselected. Ultimately, 21 studies (501 participants) were included in the final analysis (Table 1). A flow chart of the search process is illustrated in Figure 1. All selected studies were included in the qualitative analysis outlined in the next section.
Quality Assessment and Risk of Bias
A modified Downs and Black tool was used to determine the quality of the articles according to a 13-point scale (35). Up to 7 points could be awarded depending on how the study reported its findings, as well as up to 2 points for external validity and up to 4 for internal validity (bias and confounding). The quality assessment score of each article was classified as follows: 11–13: Excellent; 9–10: Good; 7–8: Fair; and ≤ 6: Poor. The level of evidence was assessed using the Oxford Centre for Evidence Based Medicine (OCEBM) system (36). The OCEBM system is hierarchical, grading studies on a scale ranging from 1 (highest level of evidence) to 5 (lowest level of evidence), and including subsections for levels 1–3. Both reviewers (BT and BW) independently scored the articles and agreed on the final score for each following a discussion.
Results
Methodological and Reporting Quality
Two randomized controlled trials (26, 27) and nineteen prospective cohort studies were included in our review (14–25, 28–34). The Oxford Level of Evidence ranged from 2b to 4b and the Downs and Black Quality Assessment revealed that there were 3 studies of good quality (26, 27, 29), 8 of fair quality (14, 16, 20, 21, 24, 25, 28, 34) and 10 of poor quality (15, 17–19, 22, 23, 30–33). Details are provided in Supplemental Table S1.
Characteristics of the Included Studies
The main findings of the included studies are summarized in Tables 2–4, stratified by the parameters measured and altitude categories. We included 1 study (17) (n = 20) conducted at moderate altitude (1,500–2,500 m), 16 (16, 18, 20–26, 28–34) (n = 422) conducted at high altitude (2,500–5,400 m) and four (14, 15, 19, 27) (n = 59) conducted at extreme altitude (>5,400 m).
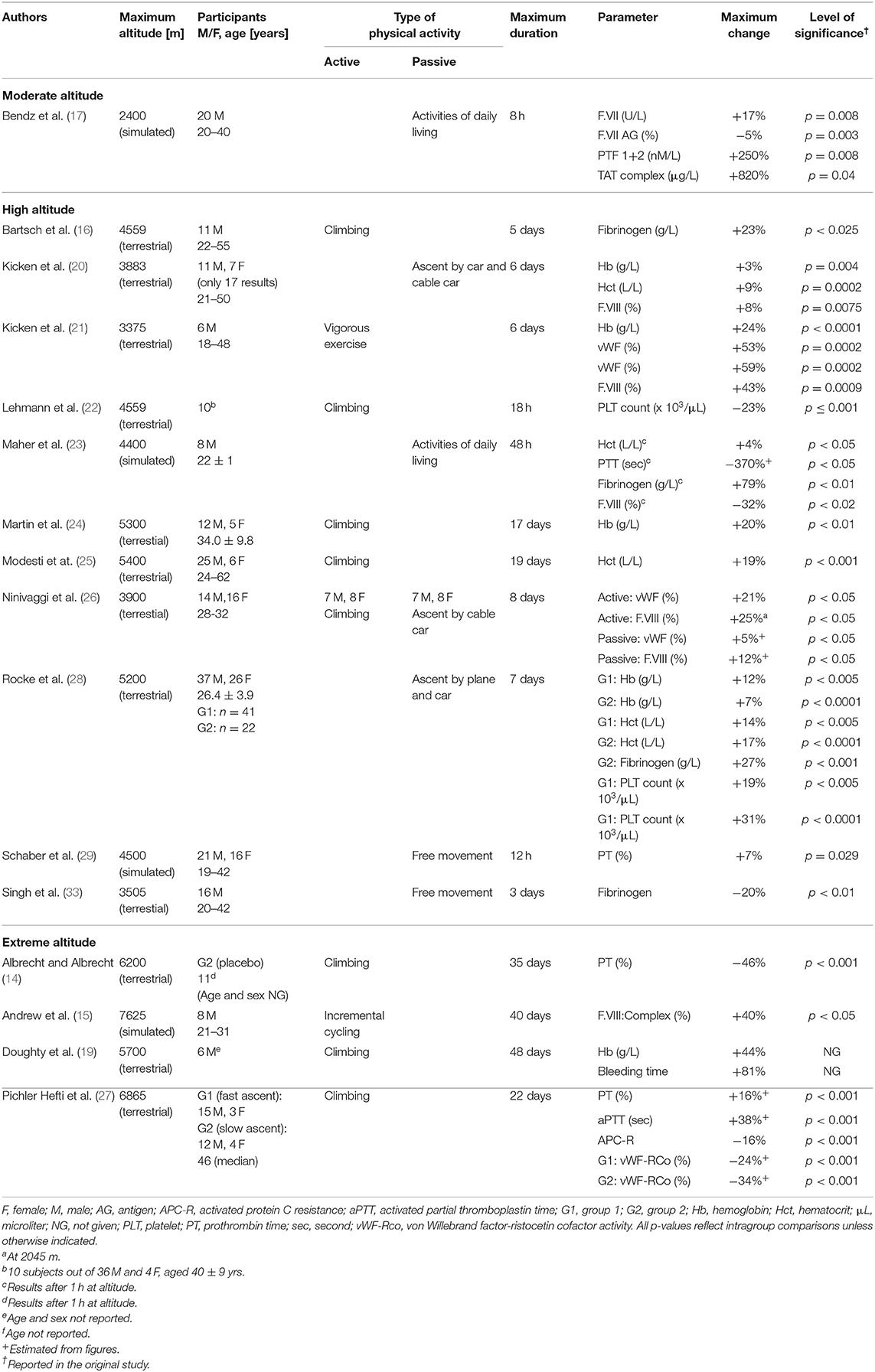
Table 2. Changes of standard coagulation parameters, coagulation factors, red blood cells and platelet count during exposure to moderate, high and extreme altitudes (16 studies, n = 388).
Four studies were conducted in the Himalayan region (19, 25, 27, 34), three (14, 24, 28) in the Andean Mountains and two in the Italian Alps (16, 22). The other seven terrestrial trials did not specify their geographical setting (20, 21, 26, 30–33).
The remaining six studies used simulated altitude: two (15, 17) in a hypobaric setting, two (18, 29) in a normobaric setting, and one (23) in a normobaric and hypobaric hypoxia setting.
Changes of Hemostasis at Moderate Altitude
A single study passively subjected 20 adults aged from 20 to 40 years to simulated altitude (17). After 8 h, increases of F.VIIa activity, F.VII antigen, prothrombin fragments 1 + 2 (PTF) and thrombin-antithrombin complex (TAT), as well as a small decrease of tissue factor pathway inhibitor (TFPI) activity and free antigen levels, have been shown.
Changes of Hemostasis at High Altitude
In total, 16 studies including 422 subjects addressed this topic. Of these studies, 11 examined changes in association with physical activity, and another eight were concerned with passive hypoxic exposure (Tables 2–4). Only one study investigated the effects of passive and active hypoxia (26). Active exposures consisted primarily of trekking or mountaineering activities, with only occasional endurance exercise. The maximum duration of altitude (or simulated altitude) exposure varied from 12 h to 30 days. The age of the subjects ranged from 18 to 62 years, with 198 being male and 71 being female. In 52 persons, sex was not reported. The number of healthy subjects ranged from 6 to 76. Hemoglobin and hematocrit were shown to increase to differing extents; one study (28) observed an increased platelet count, while another (22) reported a decreased number. Regarding global coagulation, one study (23) demonstrated an increased PTT and another (29) an increased PT. F.VIII complex has been shown to increase with exercise and activities of daily living. One study (26) reported increased F.VIII activity, in contrast to the decrease observed by Maher (23). Three of fifteen studies demonstrated increased fibrinogen (16, 23, 28); only one reported a decrease (33). Two studies showed an increase in von Willebrand factor (vWF) and vWF antigen (21, 26). In regard to fibrinolysis, a decreased clot lysis time was seen in one study (33). Zafren and co-workers demonstrated negative (qualitative) D-dimer tests (34). Four studies applied viscoelastic tests. Two studies demonstrated increased clot strength (28, 29). Data on the speed of clot formation are inconsistent, with two studies (24, 25) reporting a prolonged initiation and another a short initiation (18).
One study showed more rapid increase in thrombin generation (TG) in whole blood after 8 days (26). Another study only observed an increase in TG after 6 days (20). Regarding platelet-rich plasma (PRP), one study showed an increased time to reach the peak (20) and decreased endogenous TG (ETP) after exercise (21). Two studies (22, 28) using the PFA-100 device (Siemens Healthineers, Erlangen, Germany) demonstrated decreased closure times, while another study (28) additionally using a platelet function analyzer (Multiplate; Roche Diagnostics, Mannheim, Germany) showed an increased area under the curve. Two studies (20, 21) reported decreased spontaneous and agonist-induced platelet activation as demonstrated by decreased levels of p-selectin and the fibrinogen αIIbβ3 receptor in 24 subjects. This is contrary to the increased p-selectin levels seen in another study (22). Sharma et al. observed increased platelet adhesiveness (30). Two further studies investigating platelet function showed no significant changes with altitude (31, 32).
Changes of Hemostasis at Extreme Altitude
The four studies included on extreme altitude (n = 59) involved physical activity (mountaineering in three of them; Tables 2, 3). One study exposed the subjects to exhaustive cycling exercise at simulated altitude (15). The maximum duration of altitude/simulated altitude exposure varied from 22 to 48 days. Two of the studies reported participant age, which ranged from 21 to 31 years in the first of these studies (15) and had a median value of 46 years in the second (27). The studies included 41 males and 7 females. In 11 persons, sex was not reported. The number of subjects ranged from 6 to 34. The oldest study reported a nearly halved prothrombin time after 1 month (14). Operation Everest II demonstrated an increase in F.VIII complex after incremental bouts of cycling (15). In a later study, an increased hemoglobin and bleeding time after 48 days was seen (19). Lastly, after 3 weeks D-dimer concentrations, PT and aPTT all increased, while vWF-ristocetin cofactor activity (vWF-RCo) and activated protein C (APC) resistance decreased (27).
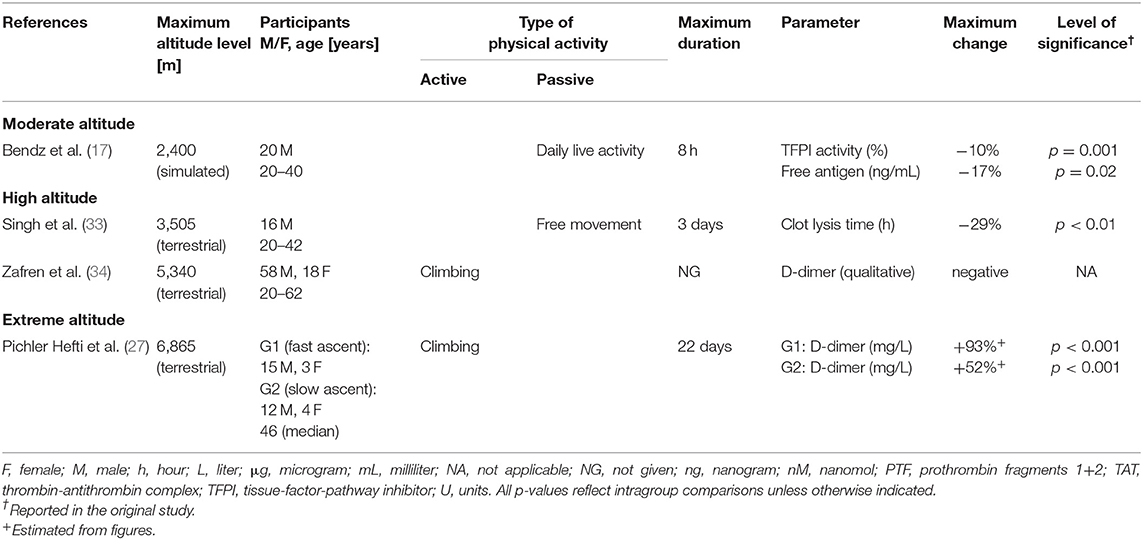
Table 3. Changes of markers for fibrinolysis during exposure to moderate, high and extreme altitudes (four studies, n = 146).
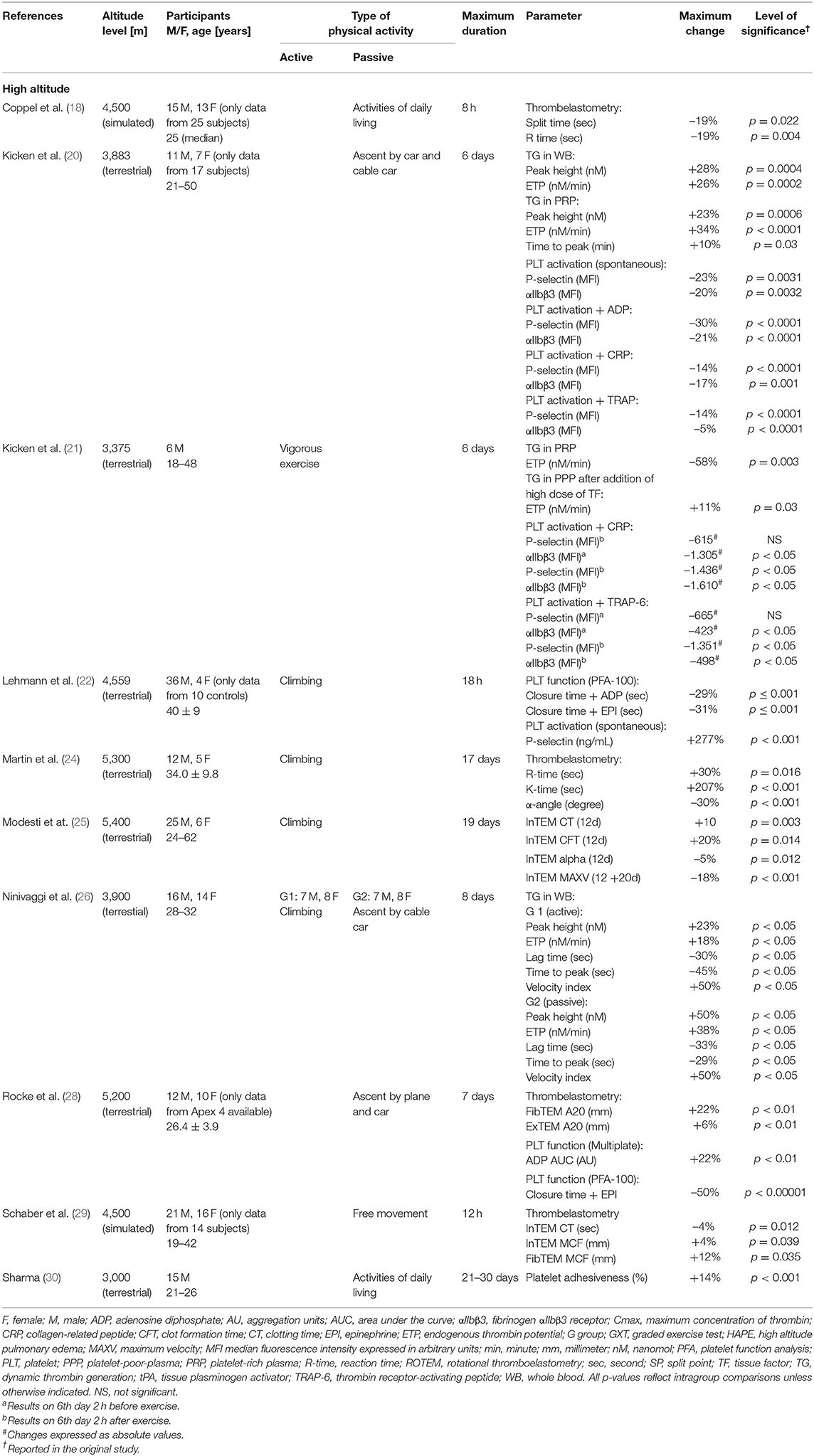
Table 4. Changes of thromboelastometry, thrombin generation and platelet function during exposure to high altitude (ten studies, n = 210).
Risk of Bias Within Studies
The multitude of protocols applied by the included studies limits comparability; for example, normobaric hypoxia and weather in a terrestrial setting represent potential confounders. The absence of blinding of participants and personnel in most of the studies may represent another source of bias. As the data of a few studies had to be estimated by manual measurements, the accuracy of the data may have been compromised. Lastly, the studied subjects were fairly young adults and predominantly male (only 102 females out of 501 subjects). These limitations have to be kept in mind when interpreting the results.
Discussion
A multitude of data on changes of hemostasis induced by hypoxic exposure, with a specific focus on prothrombotic effects, exist. However, whether there is scientific evidence of a causal relationship between hypoxic exposure, either at real or simulated altitudes, and thrombosis in healthy subjects is still a matter of debate.
Moderate Altitude and Hemostasis
At moderate altitude a single study demonstrated activation of coagulation after short-term hypobaric hypoxia, as shown by an increase in PTF and TAT (17). Both of these compounds are produced directly by thrombin activation and have been shown to indicate a risk of thrombosis (37). In addition, markers of fibrinolysis did not change during hypoxic chamber exposure. Thus, at least for moderate hypoxic conditions, there is a lack of evidence that exposure to moderate altitudes is associated with thrombogenic conditions.
High Altitude and Hemostasis
Altitude-related hemoconcentration is a phenomenon during high-altitude exposure due to a plasma volume reduction in the early phase of adaptation, along with increased erythropoiesis after several weeks and the development of polycythemia. Hemoconcentration is suggested to affect hemostasis (38). Moreover, with increasing hematocrit levels, platelets are increasingly concentrated near walls in vitro (39, 40). Our literature search revealed five trials (20, 21, 23, 25, 28) reporting slightly increased hemoglobin and hematocrit levels, while one study (16) showed no change in either parameter. Regarding global coagulation tests, the aPTT and PT results were inconsistent, with a pronounced shortening of aPTT reported after short-term hypoxia that then returned to baseline (23), along with no change of aPTT (16) or a small increase after several hours of hypoxia (29). As neither test truly reflects in vivo coagulation activity, these findings are of limited value (41). Fibrinogen plays a pivotal role in the formation of a stable clot. In contrast to a single study reporting a small drop of fibrinogen after 3 days at high altitude (33), other studies observed either slightly elevated fibrinogen levels during high-altitude exposure (16, 28) or an early increase with normalization thereafter (23). Fibrinogen remained unchanged in another study involving passive exposure (20). VWF acts as a procoagulant, mediating the adhesion of platelets to damaged vessel walls via binding both to the collagen and platelet receptor. Moreover, it mediates the protection conferred by procoagulant factor VIII. An increased plasma level is associated with a higher risk of venous thrombosis (42). In our review, vWF antigen and F.VIII showed similar reactions; VWF was increased with active exposure and exercise (21, 26), while in subjects ascending passively, hypoxia per se led to either unchanged or only minor increased levels (20, 21, 26). F.VIII activity increased to a differing extent (20, 21, 26). Greater increases were observed after strenuous exercise performed at high altitude (21) and during active ascent compared to passive ascent (26, 28). Passive ascent is associated with only minor increases in F.VIII activity (20, 21), or even with unchanged levels (16). In summary, hypoxia per se seems to increase vWF and F.VIII only slightly; physical exercise may promote a greater increase.
Two markers of thrombin activation, PFT and TAT, remained unchanged in one study (22). Newer data showed elevated TG in whole blood, but not in plasma (26). The same group reported a slight increase of F.VIII-mediated TG in whole blood in healthy inactive volunteers (20), which was balanced by decreased platelet activation. With vigorous exercise, TG in whole blood increased slightly upon arrival at a high-altitude area (and after 2 days) in platelet-poor-plasma (PPP), with a concomitant decrease of platelet activation and platelet-dependent TG (21).
Data regarding fibrinolysis largely showed a lack of changes in related parameters, such as the clot lysis time (21, 26), D-dimer level according to qualitative (34) or quantitative assay (20), and antithrombin (15, 20), alpha-2-macroglobulin, plasminogen and PC levels (15). In summary, these data support that fibrinolysis is only moderately affected by high-altitude hypoxia.
In the past few decades, platelets and platelet functions have been recognized to play a crucial role in hemostasis. Results for platelet count range from a short-term reduction after 1 day (22) to small increases after 1 week (28). All changes were within the normal laboratory ranges. With respect to platelet activity, increased platelet adhesiveness (22, 30), and platelet activation and sequestration, were reported (22). In contrast, Kicken et al. observed lower spontaneous platelet activation at high altitude compared to sea level (20). The authors stated that hypercoagulability, as reflected in the increased factor VIII-mediated TG, was balanced by decreased platelet activation. Data from the same group demonstrated a slight decrease in platelet granule release potential, and a decrease in platelet aggregation potential and platelet-dependent TG, at high altitude (21). Rocke at al. observed increased platelet reactivity, concluding that this indicates a hypoxia-induced increased in platelet reactivity, and thus a prothrombotic phenotype at altitude (28). Recently, a change in the platelet proteome in extreme hypoxia conditions (>7,000 m) was demonstrated by increased platelet reactivity in association with enhanced calpain activity in a murine model (43).
The results of viscoelastic methods are contradictory. Passively exposing 25 participants to normobaric hypoxia shortened clot initiation after 8 h (18). However, the clot formation time was still within the normal range. On the contrary, Martin et al. reported dysfunctional clot formation despite increased hemoglobin after 2 weeks (24). Modesti and co-workers reported a prolonged clot formation of the intrinsic pathway ranging within normal values (25). Schaber and co-workers demonstrated a shortening of the coagulation time and increased clot strength after 12 h, again within normal ranges (29). Lastly, Rocke showed increased clot strength after 1 week (28). However, the relationship between increased clot strength and thrombosis risk was assumed only in a high-risk surgical setting (44), in the context of recurrent ischemic events after percutaneous coronary intervention (45) and after ischemic stroke (46). Therefore, results obtained by viscoelastic methods do not support increased thrombogenicity at high altitudes within 1 week in healthy individuals traveling from low altitudes.
Extreme Altitude and Hemostasis
Studies on extreme altitudes in the literature were limited, so the influence of these altitudes on the hemostatic system is not clear. PT was demonstrated to either decrease or mildly increase after 5 weeks (14). A prolonged bleeding time, as measured by the Ivy technique after 3 weeks, is of limited value as this method is poorly reproducible (19). Recently, a prolonged aPTT in combination with PC inactivation and decreased vWF-RCo with increasing altitudes have been shown (27). The authors explained these results in terms of increased consumption of vWF due to the activated fibrinolysis. Simulated conditions of cycling exercise to exhaustion were associated with an increased F.VIII complex (15). In summary, the available data demonstrate that activation of coagulation is associated with fibrinolysis stimulation at extreme altitude. However, whether this is attributable to exercise or hypoxia per se is still unclear.
Underlying (Pathophysiological) Responses to Environmental Hypoxia
Essential for the cellular response to hypoxia is an increased stimulation of hypoxia-inducible factor (HIF) and a consequent expression of a great variety of genes (1, 47). Acute adaption to hypoxia is regulated mainly via HIF-1 which stimulates erythropoiesis and angioneogenesis. In high-altitude dwellers a less pronounced erythrocytosis, a lower pulmonary arterial blood pressure and a higher SaO2 compared to lowlanders are suggestive of this adaptive process (48). One of the pivotal changes includes a shift toward a greater HIF-2 and HIF-3 expression. Geographical differences of the same populations exist. In Tibetans a variation of EPAS1 (which encodes HIF) has been shown to be associated with lower hemoglobin levels and a decreased response to pulmonary vasoconstriction. Tibetans living at sea level have adapted by a variation of EPAS1 and a consequent hyporesponsive HIF transcriptional system (47, 49). Chronic exposure to high altitude can further result in different changes in the hematological system. Data from acquired diseases affecting hemostasis such as polycythemia vera point toward a chronic augmentation of HIF during chronic hypoxic exposure (13). Polycythemia vera in patients residing at high altitude has been shown to be associated with an increased the risk of thrombotic complications (13).
With regard to coagulation, human platelets from healthy probands have been shown to upregulate expression of HIF-2a and the antifibrinolytic PAI-1 after hypoxic exposure in vitro (50).
Despite these informations on the involvement of HIF in hypoxic adaptation, the significance of such a response on the hemostatic system for acute adaptation and short term exposure at high altitude remains to be investigated.
Strength and Limitations
One strength of our systematic review was that we only included studies providing data on healthy persons. Thus, confounding effects of pre-existing diseases can be ruled out. However, hereditary disorders of coagulation (e.g., APC resistance, PC or PS deficiency, prothrombin-complex polymorphism, etc.) were not analyzed in the selected studies at baseline, such that subjects with these conditions may have been included.
Another strength, and a novelty, of this study was our analysis of the degree of hypoxia according to altitude (moderate, high or extreme), and the sub-categorization of active and passive ascent and sojourns. Sojourns at altitude are mostly associated with physical exercise, differing in intensity and duration. However, since none of the selected studies described the exercise habits of their participants in detail, a definitive conclusion on the additive effects of hypoxia and exercise on coagulation cannot be drawn. At sea level, moderate-intensity sport does not cause hypercoagulability in healthy persons or those with lifestyle diseases. However, very intensive exertion significantly activates coagulation and platelets, and concomitantly enhances fibrinolysis, with rebalanced hemostasis seen during exercise. In contrast to hypercoagulability, which can last for several hours after exercise, fibrinolytic parameters return to baseline much earlier (51). This loss of balance immediately after strenuous exercise is suggested to be critical for persons with cardiovascular diseases and could trigger cardiovascular events (52, 53). This knowledge may be important for mountaineers and trekkers with known pre-existing cardiovascular diseases (e.g., hypertension). Since no data on the incidence of cardiovascular diseases and adverse cardiovascular events in mountaineers/trekkers at high altitudes are available, the relation between strenuous exercise under hypobaric hypoxic conditions and venous thromboembolic events (e.g., VTE, CVST, etc.) and arterial thrombosis (e.g., myocardial infarction, stroke, etc.,) needs to be investigated.
Reductions of plasma volume and hemoconcentration is a phenomenon seen early during high-altitude adaptation. Hypoxia increases red cell mass after a few weeks, leading to high-altitude polycythemia (54). Coldness, gusty winds and a high exercise load during mountaineering could further promote loss of plasma volume, leading to dehydration. However, data on the in-vivo effect of polycythemia/dehydration upon hemostasis remain equivocal. Viscoelastic tests revealed decreased clot strength with increasing amounts of red blood cells (RBCs), but this was accompanied by enhanced platelet aggregation and an increased platelet count (55). Moreover, an increased RBC count increases the endogenous thrombin potential (56). On the other hand, in-vitro hypercoagulability has been shown to be associated with low hematocrit values (57). Moreover, in-vitro studies showed that platelets were increasingly concentrated near walls with increases in hematocrit (39, 40). This may be counteracted by a decreased platelet aggregation potential at high altitude (21). To further complicate the issue, only viscoelastic and platelet function tests measure increases in hematocrit.
Sympathoadrenergic activation plays a central role in high-altitude adaptation (58). In contrast to moderate altitudes, at high and extreme altitudes the adrenergic drive persists during the entre sojourn, which is reflected in high catecholamine concentrations. Physical and psychological stress (e.g., bad weather/storms, snowfall, cold conditions, sitting in a tent with limited space, etc.,) can exacerbate adrenergic activation. Stress responses could induce a prothrombotic state characterized by autonomic and neuroendocrine dysfunction, platelet activation, dysregulation of coagulation, fibrinolysis and endothelial dysfunction (59). Since none of the studies included in this review reported such extreme conditions, it cannot be excluded that thrombogenicity is more likely under such conditions.
To rule out sex-related differences, many studies included only male volunteers. As women represented only around one quarter of all subjects, and are known to show a different hemostatic response to exercise (60, 61), the influence of gender on outcomes needs to be addressed in further studies.
One limitation of the studies included in this review is the fact that, in the older studies, the parameters measured for coagulation, fibrinolysis and platelet function are of limited methodological value for the interpretation of our findings. In addition, most studies only analyzed parts of the hemostatic system, making interpretation of the influence of hypoxia on hemostasis difficult.
Future Directions
Future trials should differentiate between the hemostatic responses to hypoxia per se and to repetitive exercise in hypoxia. These studies should include evenly distributed male and female subjects and correct for plasma volume changes. As all measurements are in vitro assays, newer technologies for coagulation monitoring could provide a deeper inside into the complex interplay of the hemostatic response to profound hypoxia in vivo.
Since the high number of trekkers and trekking tourists at high altitude remote areas we need more information on their health status before arrival and more data on adverse health outcomes during and after the tours. Thus, in order to elucidate the role of hypoxia on hemostasis, a multicenter, multinational registry is needed including data on pre-existing cardiovascular diseases and acute thrombotic and thromboembolic events.
Conclusion
At all levels of hypobaric hypoxia, i.e., from moderate to extreme altitudes, changes in the various parameters of coagulation, platelet function and fibrinolysis have been reported in the literature. However, due to the multiplicity of confounding factors at altitude (e.g., training status, duration and intensity of exercise, fluid status, mental stress, etc.,), there is no consensus on the role of hypoxia alone in these changes. Referring to a hypothesis suggested in the literature, hypoxia itself could be the trigger for a thrombogenic state. Therefore, we cannot support the assumption that hypoxia occurring in mountainous regions is the primary trigger for hypercoagulability and an increased risk of thromboembolic disorders, at least in healthy persons.
Data Availability Statement
The original contributions presented in the study are included in the article/Supplementary Material, further inquiries can be directed to the corresponding author.
Author Contributions
WS and DF: conceptualization of the study. WS and CB: methodology. BT, BW, WS, and CB: data analysis. WS, CB, and DF: supervision. BT, BW, and WS: writing—original draft. BT, BW, WS, CB, and DF: writing—review and editing. All authors contributed to the article and approved the submitted version.
Conflict of Interest
CB and WS were employed by Private University for Health Sciences, Medical Informatics and Technology UMIT, Hall i.T. and Tirol Kliniken GmbH.
The remaining authors declare that the research was conducted in the absence of any commercial or financial relationships that could be construed as a potential conflict of interest.
Publisher's Note
All claims expressed in this article are solely those of the authors and do not necessarily represent those of their affiliated organizations, or those of the publisher, the editors and the reviewers. Any product that may be evaluated in this article, or claim that may be made by its manufacturer, is not guaranteed or endorsed by the publisher.
Acknowledgments
We are thankful to our native English proofreader (https://www.theukproofreaders.com/) for his support in the final preparation of this work.
Supplementary Material
The Supplementary Material for this article can be found online at: https://www.frontiersin.org/articles/10.3389/fcvm.2022.813550/full#supplementary-material
Supplementary Table S1. Oxford Level of Evidence scale and modified Downs and Black Quality Assessment results.
Abbreviations
APC, activated protein C; CVST, cerebral venous sinus thrombosis; HACE, high-altitude cerebral edema; HAPE, high-altitude pulmonary edema; HIF, hypoxia inducible factors; PAE, pulmonary arterial embolism; PTF, prothrombin fragments 1 + 2; PC, protein C; PS, protein S; RBC, red blood cell; ROTEM, rotational thrombroelastometry; TAT, thrombin-antithrombin complex; TEG, thrombelastography; TFPI, tissue factor pathway inhibitor; TG, thrombin generation; VWF-RCo, von Willebrand Factor-ristocetin cofactor activity; VTE, venous thromboembolism.
References
1. Prabhakar NR, Semenza GL. Adaptive and maladaptive cardiorespiratory responses to continuous and intermittent hypoxia mediated by hypoxia-inducible factors 1 and 2. Physiol Rev. (2012) 92:967–1003. doi: 10.1152/physrev.00030.2011
2. Luks AM, Swenson ER, Bartsch P. Acute high-altitude sickness. Eur Respir Rev. (2017) 26:160096. doi: 10.1183/16000617.0096-2016
3. Gupta N, Ashraf MZ. Exposure to high altitude: a risk factor for venous thromboembolism? Semin Thromb Hemost. (2012) 38:156–63. doi: 10.1055/s-0032-1301413
4. Pandey P, Lohani B, Murphy H. Pulmonary Embolism masquerading as high altitude pulmonary edema at high altitude. High Alt Med Biol. (2016) 17:353–8. doi: 10.1089/ham.2016.0008
5. Zavanone C, Panebianco M, Yger M, Borden A, Restivo D, Angelini C, et al. Cerebral venous thrombosis at high altitude: a systematic review. Rev Neurol. (2017) 173:189–93. doi: 10.1016/j.neurol.2016.11.004
6. Syed MJ, Khatri IA, Alamgir W, Wasay M. Stroke at moderate and high altitude. High Alt Med Biol. (2021). doi: 10.1089/ham.2021.0043. [Epub ahead of print].
7. Trunk AD, Rondina MT, Kaplan DA. Venous thromboembolism at high altitude: our approach to patients at risk. High Alt Med Biol. (2019) 20:331–6. doi: 10.1089/ham.2019.0049
8. Hassan WU, Syed MJ, Alamgir W, Awan S, Bell SM, Majid A, et al. Cerebral venous thrombosis at high altitude: analysis of 28 cases. Cerebrovasc Dis. (2019) 48:184–92. doi: 10.1159/000504504
9. Khanal P, Thapa L, Shrestha AM, Bhattarai S, Sapkota D, Sharma N, et al. Cerebral venous sinus thrombosis during everest expedition: a case report and review of the literature. Case Rep Neurol Med. (2016) 2016:8314040. doi: 10.1155/2016/8314040
10. Khatri IA, AlKawi A, Ilyas A, Ilyas MS. Unusual causes of cerebral venous thrombosis. J Pak Med Assoc. (2006) 56:501–6.
11. Boulos P, Kouroukis C, Blake G. Superior sagittal sinus thrombosis occurring at high altitude associated with protein C deficiency. Acta Haematol. (1999) 102:104–6. doi: 10.1159/000040980
12. Saadatnia M, Fatehi F, Basiri K, Mousavi SA, Mehr GK. Cerebral venous sinus thrombosis risk factors. Int J Stroke. (2009) 4:111–23. doi: 10.1111/j.1747-4949.2009.00260.x
13. Gangaraju R, Song J, Kim SJ, Tashi T, Reeves BN, Sundar KM, et al. Thrombotic, inflammatory, and HIF-regulated genes and thrombosis risk in polycythemia vera and essential thrombocythemia. Blood Adv. (2020) 4:1115–30. doi: 10.1182/bloodadvances.2019001379
14. Albrecht E, Albrecht H. Metabolism and hematology at high altitude and the effect of drugs on acclimatization. Fed Proc. (1969) 28:1118–23.
15. Andrew M, O'Brodovich H, Sutton J. Operation everest II: coagulation system during prolonged decompression to 282 torr. J Appl Physiol. (1987) 63:1262–7. doi: 10.1152/jappl.1987.63.3.1262
16. Bartsch P, Lammle B, Huber I, Haeberli A, Vock P, Oelz O, et al. Contact phase of blood coagulation is not activated in edema of high altitude. J Appl Physiol. (1989) 67:1336–40. doi: 10.1152/jappl.1989.67.4.1336
17. Bendz B, Rostrup M, Sevre K, Andersen TO, Sandset PM. Association between acute hypobaric hypoxia and activation of coagulation in human beings. Lancet. (2000) 356:1657–8. doi: 10.1016/S0140-6736(00)03165-2
18. Coppel J, Gilbert-Kawai E, Bottomley T, Sugarman J, Martin D. The effect of normobaric hypoxic exposure on coagulation as measured by thromboelastography. Thromb Res. (2019) 184:105–9. doi: 10.1016/j.thromres.2019.10.032
20. Kicken CH, Ninivaggi M, Konings J, Moorlag M, Huskens D, Remijn JA, et al. Hypobaric hypoxia causes elevated thrombin generation mediated by FVIII that is balanced by decreased platelet activation. Thromb Haemost. (2018) 118:883–92. doi: 10.1055/s-0038-1641566
21. Kicken CH, van der Vorm LN, Zwaveling S, Schoenmaker E, Remijn JA, Huskens D, et al. A hypoxic environment attenuates exercise-induced procoagulant changes due to decreased platelet activation. TH Open. (2019) 3:e216–26. doi: 10.1055/s-0039-1692991
22. Lehmann T, Mairbaurl H, Pleisch B, Maggiorini M, Bartsch P, Reinhart WH. Platelet count and function at high altitude and in high-altitude pulmonary edema. J Appl Physiol. (2006) 100:690–4. doi: 10.1152/japplphysiol.00991.2005
23. Maher JT, Levine PH, Cymerman A. Human coagulation abnormalities during acute exposure to hypobaric hypoxia. J Appl Physiol. (1976) 41:702–7. doi: 10.1152/jappl.1976.41.5.702
24. Martin DS, Pate JS, Vercueil A, Doyle PW, Mythen MG, Grocott MP, et al. Reduced coagulation at high altitude identified by thromboelastography. Thromb Haemost. (2012) 107:1066–71. doi: 10.1160/TH12-01-0004
25. Modesti PA, Rapi S, Paniccia R, Bilo G, Revera M, Agostoni P, et al. Index measured at an intermediate altitude to predict impending acute mountain sickness. Med Sci Sports Exerc. (2011) 43:1811–8. doi: 10.1249/MSS.0b013e31821b55df
26. Ninivaggi M, de Laat M, Lance MM, Kicken CH, Pelkmans L, Bloemen S, et al. Hypoxia induces a prothrombotic state independently of the physical activity. PLoS ONE. (2015) 10:e0141797. doi: 10.1371/journal.pone.0141797
27. Hefti JP, Risch L, Hefti U, Scharrer I, Risch G, Merz TM, et al. Changes of coagulation parameters during high altitude expedition. Swiss Med Wkly. (2010) 140:111–7. doi: 10.7892/boris.3055
28. Rocke AS, Paterson GG, Barber MT, Jackson AIR, Main SE, Stannett C, et al. Thromboelastometry and platelet function during acclimatization to high altitude. Thromb Haemost. (2018) 118:63–71. doi: 10.1160/TH17-02-0138
29. Schaber M, Leichtfried V, Fries D, Wille M, Gatterer H, Faulhaber M, et al. Influence of acute normobaric hypoxia on hemostasis in volunteers with and without acute mountain sickness. Biomed Res Int. (2015) 2015:593938. doi: 10.1155/2015/593938
30. Sharma SC. Platelet adhesiveness in temporary residents of high altitude. Thromb Res. (1981) 21:685–7. doi: 10.1016/0049-3848(81)90271-1
31. Sharma SC. Platelet count and adhesiveness on induction to high altitude by air and road. Int J Biometeorol. (1982) 26:219–24. doi: 10.1007/BF02184937
32. Sharma SC, Hoon RS. Platelet adhesiveness on acute induction to high altitude. Thromb Res. (1978) 13:725–32. doi: 10.1016/0049-3848(78)90178-0
33. Singh I, Chohan IS, Mathew NT. Fibrinolytic activity in high altitude pulmonary oedema. Indian J Med Res. (1969) 57:210–7.
34. Zafren K, Feldman J, Becker RJ, Williams SR, Weiss EA, Deloughery T. D-dimer is not elevated in asymptomatic high altitude climbers after descent to 5340 m: the Mount Everest Deep Venous Thrombosis Study (Ev-DVT). High Alt Med Biol. (2011) 12:223–7. doi: 10.1089/ham.2010.1101
35. Downs SH, Black N. The feasibility of creating a checklist for the assessment of the methodological quality both of randomised and non-randomised studies of health care interventions. J Epidemiol Community Health. (1998) 52:377–84. doi: 10.1136/jech.52.6.377
36. Centre, for Evidence-Based Medicine (CEBM) UoO. Oxford Centre for Evidence Based Medicine: Levels of Evidence. Available online at: https://www.cebm.ox.ac.uk/resources/levels-of-evidence/oxford-centre-for-evidence-based-medicine-levels-of-evidence-march-2009.
37. Ota S, Wada H, Abe Y, Yamada E, Sakaguchi A, Nishioka J, et al. Elevated levels of prothrombin fragment 1 + 2 indicate high risk of thrombosis. Clin Appl Thromb Hemost. (2008) 14:279–85. doi: 10.1177/1076029607309176
38. Goldsmith HL, Kaufer ES, McIntosh FA. Effect of hematocrit on adenosine diphosphate-induced aggregation of human platelets in tube flow. Biorheology. (1995) 32:537–52. doi: 10.3233/BIR-1995-32503
39. Escolar G, Garrido M, Mazzara R, Castillo R, Ordinas A. Experimental basis for the use of red cell transfusion in the management of anemic-thrombocytopenic patients. Transfusion. (1988) 28:406–11. doi: 10.1046/j.1537-2995.1988.28588337325.x
40. Uijttewaal WS, Nijhof EJ, Bronkhorst PJ, Den Hartog E, Heethaar RM. Near-wall excess of platelets induced by lateral migration of erythrocytes in flowing blood. Am J Physiol. (1993) 264:H1239–44. doi: 10.1152/ajpheart.1993.264.4.H1239
41. Tanaka KA, Key NS, Levy JH. Blood coagulation: hemostasis and thrombin regulation. Anesth Analg. (2009) 108:1433–46. doi: 10.1213/ane.0b013e31819bcc9c
42. Rietveld IM, Lijfering WM, le Cessie S, Bos MHA, Rosendaal FR, Reitsma PH, et al. High levels of coagulation factors and venous thrombosis risk: strongest association for factor VIII and von willebrand factor. J Thromb Haemost. (2019) 17:99–109. doi: 10.1111/jth.14343
43. Tyagi T, Ahmad S, Gupta N, Sahu A, Ahmad Y, Nair V, et al. Altered expression of platelet proteins and calpain activity mediate hypoxia-induced prothrombotic phenotype. Blood. (2014) 123:1250–60. doi: 10.1182/blood-2013-05-501924
44. Dai Y, Lee A, Critchley LA, White PF. Does thromboelastography predict postoperative thromboembolic events? A systematic review of the literature. Anesth Analg. (2009) 108:734–42. doi: 10.1213/ane.0b013e31818f8907
45. Gurbel PA, Bliden KP, Guyer K, Cho PW, Zaman KA, Kreutz RP, et al. Platelet reactivity in patients and recurrent events post-stenting: results of the PREPARE POST-STENTING Study. J Am Coll Cardiol. (2005) 46:1820–6. doi: 10.1016/j.jacc.2005.07.041
46. Yao X, Dong Q, Song Y, Wang Y, Deng Y, Li Y. Thrombelastography maximal clot strength could predict one-year functional outcome in patients with ischemic stroke. Cerebrovasc Dis. (2014) 38:182–90. doi: 10.1159/000365652
47. Dzhalilova D, Makarova O. Differences in tolerance to hypoxia: physiological, biochemical, and molecular-biological characteristics. Biomedicines. (2020) 8:428. doi: 10.3390/biomedicines8100428
48. Li C, Li X, Liu J, Fan X, You G, Zhao L, et al. Investigation of the differences between the Tibetan and Han populations in the hemoglobin-oxygen affinity of red blood cells and in the adaptation to high-altitude environments. Hematology. (2018) 23:309–13. doi: 10.1080/10245332.2017.1396046
49. Peng Y, Cui C, He Y, Ouzhuluobu Zhang H, Yang D, et al. Down-regulation of epas1 transcription and genetic adaptation of tibetans to high-altitude hypoxia. Mol Biol Evol. (2017) 34:818–30. doi: 10.1093/molbev/msw280
50. Chaurasia SN, Kushwaha G, Kulkarni PP, Mallick RL, Latheef NA, Mishra JK, et al. platelet HIF-2alpha promotes thrombogenicity through PAI-1 synthesis and extracellular vesicle release. Haematologica. (2019) 104:2482–92. doi: 10.3324/haematol.2019.217463
51. Kicken CH, Miszta A, Kelchtermans H, De Laat B. Hemostasis during Extreme Exertion. Semin Thromb Hemost. (2018) 44:640–50. doi: 10.1055/s-0038-1639502
52. Braschi A. Acute exercise-induced changes in hemostatic and fibrinolytic properties: analogies, similarities, and differences between normotensive subjects and patients with essential hypertension. Platelets. (2019) 30:675–89. doi: 10.1080/09537104.2019.1615611
53. Olsen LN, Fischer M, Evans PA, Gliemann L, Hellsten Y. Does exercise influence the susceptibility to arterial thrombosis? An integrative perspective. Front Physiol. (2021) 12:636027. doi: 10.3389/fphys.2021.636027
54. Rasmussen P, Siebenmann C, Diaz V, Lundby C. Red cell volume expansion at altitude: a meta-analysis and monte carlo simulation. Med Sci Sports Exerc. (2013) 45:1767–72. doi: 10.1249/MSS.0b013e31829047e5
55. Bochsen L, Johansson PI, Kristensen AT, Daugaard G, Ostrowski SR. The influence of platelets, plasma and red blood cells on functional haemostatic assays. Blood Coagul Fibrinolysis. (2011) 22:167–75. doi: 10.1097/MBC.0b013e3283424911
56. Peyrou V, Lormeau JC, Herault JP, Gaich C, Pfliegger AM, Herbert JM. Contribution of erythrocytes to thrombin generation in whole blood. Thromb Haemost. (1999) 81:400–6. doi: 10.1055/s-0037-1614486
57. Iselin BM, Willimann PF, Seifert B, Casutt M, Bombeli T, Zalunardo MP, et al. Isolated reduction of haematocrit does not compromise in vitro blood coagulation. Br J Anaesth. (2001) 87:246–9. doi: 10.1093/bja/87.2.246
58. Richalet JP. Physiological and clinical implications of adrenergic pathways at high altitude. Adv Exp Med Biol. (2016) 903:343–56. doi: 10.1007/978-1-4899-7678-9_23
59. Sandrini L, Ieraci A, Amadio P, Zara M, Barbieri SS. Impact of acute and chronic stress on thrombosis in healthy individuals and cardiovascular disease patients. Int J Mol Sci. (2020) 21:7818. doi: 10.3390/ijms21217818
60. Huskens D, Roest M, Remijn JA, Konings J, Kremers RM, Bloemen S, et al. Strenuous exercise induces a hyperreactive rebalanced haemostatic state that is more pronounced in men. Thromb Haemost. (2016) 115:1109–19. doi: 10.1160/TH15-10-0821
Keywords: hypoxia, altitude, hemostasis, coagulation, fibrinolysis, thrombin generation
Citation: Treml B, Wallner B, Blank C, Fries D and Schobersberger W (2022) The Influence of Environmental Hypoxia on Hemostasis—A Systematic Review. Front. Cardiovasc. Med. 9:813550. doi: 10.3389/fcvm.2022.813550
Received: 11 November 2021; Accepted: 24 January 2022;
Published: 18 February 2022.
Edited by:
Grzegorz Bilo, University of Milano Bicocca, ItalyReviewed by:
Josef Prchal, The University of Utah, United StatesFriedrich Luft, Charite University Medicine Berlin, Germany
Copyright © 2022 Treml, Wallner, Blank, Fries and Schobersberger. This is an open-access article distributed under the terms of the Creative Commons Attribution License (CC BY). The use, distribution or reproduction in other forums is permitted, provided the original author(s) and the copyright owner(s) are credited and that the original publication in this journal is cited, in accordance with accepted academic practice. No use, distribution or reproduction is permitted which does not comply with these terms.
*Correspondence: Bernd Wallner, bernd.wallner@tirol-kliniken.at