Myosteatosis Significantly Predicts Persistent Dyspnea and Mobility Problems in COVID-19 Survivors
- 1Division of Immunology, Transplantation and Infectious Diseases, IRCCS San Raffaele Scientific Institute, Milan, Italy
- 2School of Medicine, Vita-Salute San Raffaele University, Milan, Italy
- 3Clinical and Experimental Radiology Unit, Experimental Imaging Center, IRCCS San Raffaele Scientific Institute, Milan, Italy
- 4Department of Translational and Precision Medicine, Sapienza University of Rome, Rome, Italy
- 5Department of Human Sciences and Promotion of the Quality of Life, San Raffaele Roma Open University, Rome, Italy
- 6Department of Endocrinology, Nutrition and Metabolic Diseases, IRCCS MultiMedica, Milan, Italy
Background: Persistent symptoms including dyspnea and functional impairment are common in COVID-19 survivors. Poor muscle quality (myosteatosis) associates with poor short-term outcomes in COVID-19 patients. The aim of this observational study was to assess the relationship between myosteatosis diagnosed during acute COVID-19 and patient-reported outcomes at 6 months after discharge.
Methods: Myosteatosis was diagnosed based on CT-derived skeletal muscle radiation attenuation (SM-RA) measured during hospitalization in 97 COVID-19 survivors who had available anthropometric and clinical data upon admission and at the 6-month follow-up after discharge. Dyspnea in daily activities was assessed using the modified Medical Research Council (mMRC) scale for dyspnea. Health-related quality of life was measured using the European quality of life questionnaire three-level version (EQ-5D-3L).
Results: Characteristics of patients with (lowest sex- and age-specific tertile of SM-RA) or without myosteatosis during acute COVID-19 were similar. At 6 months, patients with myosteatosis had greater rates of obesity (48.4 vs. 27.7%, p = 0.046), abdominal obesity (80.0 vs. 47.6%, p = 0.003), dyspnea (32.3 vs. 12.5%, p = 0.021) and mobility problems (32.3 vs. 12.5%, p = 0.004). Myosteatosis diagnosed during acute COVID-19 was the only significant predictor of persistent dyspnea (OR 3.19 [95% C.I. 1.04; 9.87], p = 0.043) and mobility problems (OR 3.70 [95% C.I. 1.25; 10.95], p = 0.018) at 6 months at logistic regression adjusted for sex, age, and BMI.
Conclusion: Myosteatosis diagnosed during acute COVID-19 significantly predicts persistent dyspnea and mobility problems at 6 months after hospital discharge independent of age, sex, and body mass.
Clinical Trial Registration: [www.ClinicalTrials.gov], identifier [NCT04318366].
Introduction
Coronavirus disease 2019 (COVID-19) poses a severe burden on survivors, with clinically relevant nutritional and functional impairments. At hospital discharge, more than 70% of patients who survive critical COVID-19 report problems in mobility and in conducting their usual activities (1). At 6 months after hospital discharge, a significant proportion of COVID-19 survivors have impaired functional status (2) and persistent symptoms including dyspnea, fatigue, and muscle weakness (3). Patients with more severe illness during the acute phase are those with more troublesome sequelae (4). However, even patients with mild disease experience persisting symptoms after SARS-CoV-2 infection (5, 6). Few studies have investigated factors associated with symptom persistence in the long-term. Frailty, multiple symptoms at disease onset, female sex, endothelial dysfunction, overweight/obesity, and pre-existing comorbidities have been suggested as potential predictors (4, 7–9). Reduced muscle mass and quality might also contribute to persistent functional impairment. In non-COVID-19 critically ill patients, pre-existing low muscle mass independently predicts poor functional status at 12 months after intra−abdominal sepsis (10). Muscle quality is even more related to muscle function or strength as compared with muscle mass (11). The term muscle quality generally refers to the ability of skeletal muscle to effectively perform its functions, the most prominent being force production. Muscle quality depends on several determinants, including fiber type and distribution, muscle architecture, neuromuscular activation, the amount of fibrous tissue and the extent of fat infiltration, i.e., myosteatosis (12). The latter has emerged as an important biomarker and a priority research focus, being an independent risk factor for metabolic dysfunction, hip fractures, disability, hospitalization, mortality, and poor outcomes in severe illness and surgery (13, 14). Computed tomography (CT)-derived skeletal muscle radiodensity, also known as skeletal muscle radiation attenuation (SM-RA), is a reliable marker of myosteatosis (15). Low CT-derived SM-RA, which indicates myosteatosis, is independently associated with higher 6-month mortality in critically ill patients (16). In surgical patients, low SM-RA predicts postoperative complications or mortality (17–21), being a more reliable predictor than muscle size (20, 21). Furthermore, low trunk SM-RA is strongly associated with reduced functional capacity in both inpatients and outpatients (22–24).
Recent evidence indicates that myosteatosis was diagnosed by opportunistic CT-derived SM-RA associates with poor short-term outcomes in COVID-19 patients (25–28). It is not known whether poor muscle quality, as reflected by the presence of myosteatosis, influences clinical outcomes of COVID-19 survivors in the medium term. Therefore, the aim of this study was to assess the relationship between myosteatosis detected during acute COVID-19 and patient reported outcomes at 6 months after discharge.
Materials and Methods
Study Design
This analysis is part of the COVID-BioB study, a large observational investigation performed at San Raffaele University Hospital in Milan, Italy. The study protocol was approved by the IRCCS San Raffaele Hospital Ethics Committee (protocol no. 34/int/2020) and was registered on ClinicalTrials.gov (NCT04318366). The study was conducted in accordance with the World Medical Association’s Declaration of Helsinki. All patients provided a signed informed consent. Full description of patient management and clinical protocols were previously published (29, 30). The reporting of this study conforms to the Strengthening the Reporting of Observational Studies in Epidemiology (STROBE) Statement for cohort studies (31).
Participants
All patients aged ≥ 18 years admitted to the Emergency Department (ED) at San Raffaele University Hospital with confirmed SARS-CoV-2 infection were consecutively enrolled in the COVID-BioB study. Confirmed infection was defined as positive real-time reverse-transcriptase polymerase chain reaction (RT-PCR) from a nasal and/or throat swab together with signs, symptoms, and radiological findings suggestive of COVID-19 pneumonia. Only COVID-19 survivors hospitalized during the first pandemic wave who underwent a CT scan and had available anthropometric data upon admission and at the 6-month follow-up were included in the present analysis. Patients admitted for other reasons and subsequently diagnosed with superimposed SARS-CoV-2 infection were excluded.
Assessments
Data were collected from medical chart review or directly by patient interview and entered in a dedicated electronic case record form (eCRF) specifically developed for the COVID-BioB study. Prior to the analysis, data were cross-checked with medical charts and verified by data managers and clinicians for accuracy. The following variables were collected for all patients: age, sex, body mass index [BMI, calculated as the ratio of weight in kilograms (kg) divided by height in squared meters], laboratory parameters on hospital admission, comorbidities (including history of hypertension, diabetes mellitus, ischaemic heart disease, and active malignancy), length of stay (LoS) and treatment intensity [admission to the intensive care unit (ICU)]. The 6-month follow-up visit included a complete internal medicine assessment (collection of medical history, physical examination), and measurement of anthropometrics (body weight measured to the nearest 0.1 kg using a balance beam scale, height measured to the nearest 0.1 cm using a wall-mounted stadiometer, waist circumference measurements taken around the abdomen at the level of the umbilicus). Abdominal obesity was defined as a waist circumference ≥ 88 cm in women and ≥ 102 cm in men (32).
Dyspnea in daily activities was assessed using the modified Medical Research Council (mMRC) scale for dyspnea. Patients with a mMRC score ≥ 2 were classified as having dyspnea (33, 34).
Health-related quality of life was measured using the European quality of life questionnaire three-level version (EQ-5D-3L) (35). The EQ-5D-3L comprises five dimensions (mobility, self-care, usual activities, pain/discomfort, and anxiety/depression) and a visual analog scale (EQ VAS). Each dimension has 3 levels, labeled 1–3: no problems, some problems, and extreme problems. For the present analysis, the EQ-5D levels were dichotomized into “no problems” (level 1) and “any problems” (levels 2 and 3). The EQ VAS records the patient’s self-rated health on a vertical visual analog scale where the endpoints are labeled “best imaginable health state” and “worst imaginable health state.”
CT Scan Protocol and Image Analysis
Non-contrast chest CT scans were performed in a dedicated suite easily accessible via assigned elevators and paths, on a 64-slice scanner (LightSpeed V CT, GE Healthcare) in supine position, during inspiratory breath hold. CT scan parameters were as follows: 120 kV tube voltage, automatic tube current modulation 150–550 mA, 0⋅4 s rotation time, pitch 1⋅375 mm/rot, 64 × 0⋅625 mm detector collimation. Images were reconstructed at 1⋅25 and 3 mm slice thickness with sharp and medium-soft kernel, respectively, for lung and mediastinum evaluation. The lung and mediastinal window width and level were set as 1,500/–700 Hounsfield Units (HU) and 350/40 HU, respectively. Mean dose length product was 438 ± 153 mGy⋅cm.
SM-RA was measured directly analyzing CT images on the picture archiving and communication system (PACS) on axial images with mediastinal filter reconstruction. Cross-sectional areas (CSA) of the paravertebral skeletal muscle mass on both sides of the spine including the erector spinae, longissimus thoracis, spinalis thoracis, and iliocostalis lumborum muscles were manually drawn at T11-T12 level. To estimate muscle mass, the paravertebral skeletal muscle index (pSMI) was calculated as the bilateral cross-sectional area of paravertebral skeletal muscles normalized by the height squared (cm2/m2) (36, 37). The SM-RA values relative to each ROI were averaged to obtain a single value for each patient. The average SM-RA values reflect the composition of muscle, with lower mean values due to the intrinsic difference in HU values between muscle (-29 to + 150 HU) (38) and fat (−30 to −190 HU) (39) indicating higher fat content. Therefore, the lower the SM-RA, the higher the degree of myosteatosis (14). As univocal cut-off values based on paraspinal SM-RA for diagnosing thoracic myosteatosis are not available (13), we identified our own cut-off values based on tertiles stratified by age (≥ or < 60 years) and sex. A similar approach has been adopted by other authors for samples of similar size (28, 40). Cutoff values for defining myosteatosis were set at the lowest tertiles of SM-RA.
Statistical Analysis
Descriptive statistics were obtained for all study variables. Continuous variables were expressed as medians (25th–75th percentile). Categorical variables were summarized as counts and percentages. Categorical variables were compared using the Fisher exact test or χ2-test, as appropriate, and continuous variables were compared using the Mann-Whitney U-test. Binomial logistic regression was used to assess the effect of myosteatosis on the likelihood of persistent dyspnea and problems in the EQ-5D dimensions at 6 months after discharge, with age, sex, and BMI as covariates. Multicollinearity was assessed using variance inflation factor (VIF). All VIFs were < 5, ranging between 1.31 and 1.64. All statistical tests were two−tailed, with p < 0.05 considered significant. No sample size calculation was performed for the present analysis; the sample size was established by the time window of the study. Missing data were not imputed. Statistical analyses were performed using IBM SPSS Statistics (IBM SPSS Statistics for Windows, Version 22.0. Armonk, NY: IBM Corp.).
Results
Patient Characteristics
Between February 25th, 2020 and April 19th, 2020, 652 patients with confirmed SARS-CoV-2 infection were hospitalized at San Raffaele University Hospital, of which 97 underwent a CT scan and had baseline and 6-month clinical and anthropometric data available for analyses (Supplementary Figure 1). Median age was 60.0 years (54.5; 69.5). Most patients were males (79.5%) and of Caucasian ethnicity (88.7%). Overall, nearly one third (32%) of patients had obesity. The most common comorbidity was arterial hypertension (38.5%), followed by DM (10.3%) and coronary heart disease (8.2%). Overall, 17.5% of patients required admission to the ICU during the hospital stay. Median length of stay (LoS) was 15.0 (10.0; 28.0) days.
Comparison Between Patients With and Without Myosteatosis
Median time from admission to CT scan was 3.0 (1.0; 5.5) days. SM-RA cut-offs for defining myosteatosis were 48.90 and 49.98 HU in females and males younger than 60 years, and 18.3 and 36.5 HU in females and males aged 60 years or older, respectively. Figure 1 shows the cross-sectional CT images of a 30-year-old man with high SM-RA and a 72-year-old woman with low SM-RA. A comparison between patients with or without myosteatosis is provided in Table 1. Except for the lymphocyte count, which was significantly lower, and a tendency toward a higher prevalence of obesity in those with myosteatosis, patient characteristics relative to the hospital stay were similar between groups. There was no difference in the proportion of patients admitted to the ICU. By design, SM-RA was significantly lower in patients with myosteatosis. After a median of 184.0 (176.0; 192.0) days since discharge, median BMI and the prevalence of obesity were significantly higher in patients with myosteatosis, as was the proportion of patients with abdominal obesity (Table 2). Although self-rated health (EQ VAS) was similar between groups, in the group with myosteatosis there were more patients complaining of dyspnea and mobility problems, as compared with participants without myosteatosis (Figure 2). The proportion of patients reporting issues in the other EQ5D domains was numerically greater in the group with myosteatosis, the difference in the self-care dimension being borderline significant (19.4 vs. 4.8%, p = 0.055).
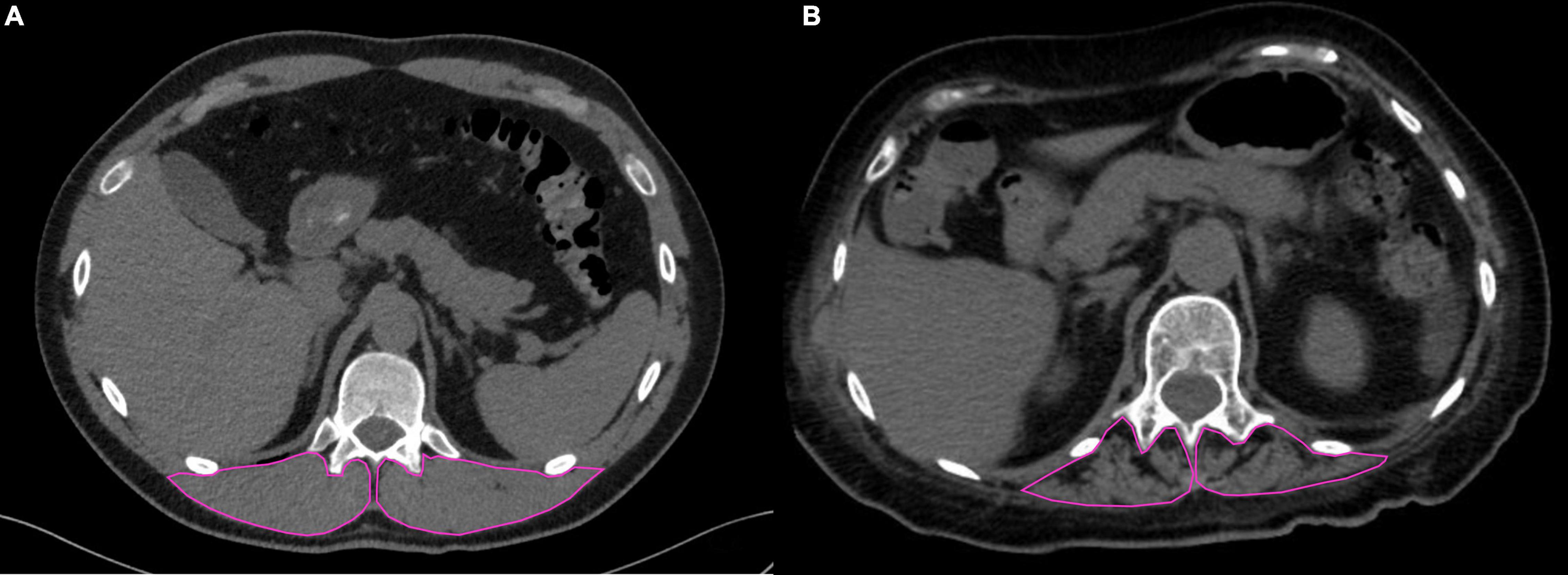
Figure 1. Chest CT images at the T12 level in two patients with COVID-19 pneumonia. In (A) (30-year-old man), the skeletal muscle area segmented at the T12 level is characterized by normal mass and high mean SM-RA (56.5 HU); in (B) (72-year-old woman), impaired muscle status is evident, with fat infiltration and low mean SM-RA (11.1 HU).
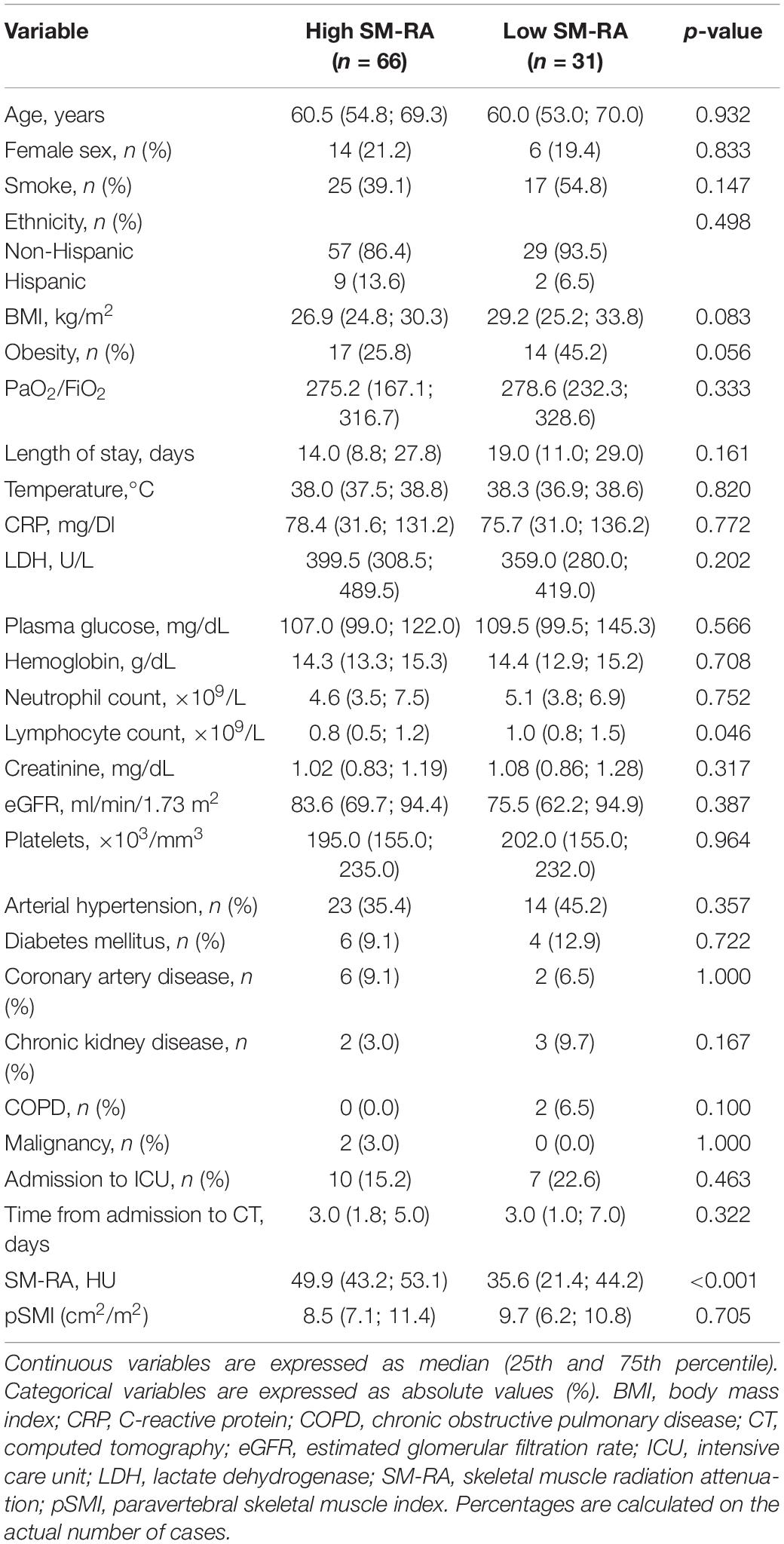
Table 1. Comparison between patients with (low SM-RA) or without (high SM-RA) myosteatosis during acute COVID-19.
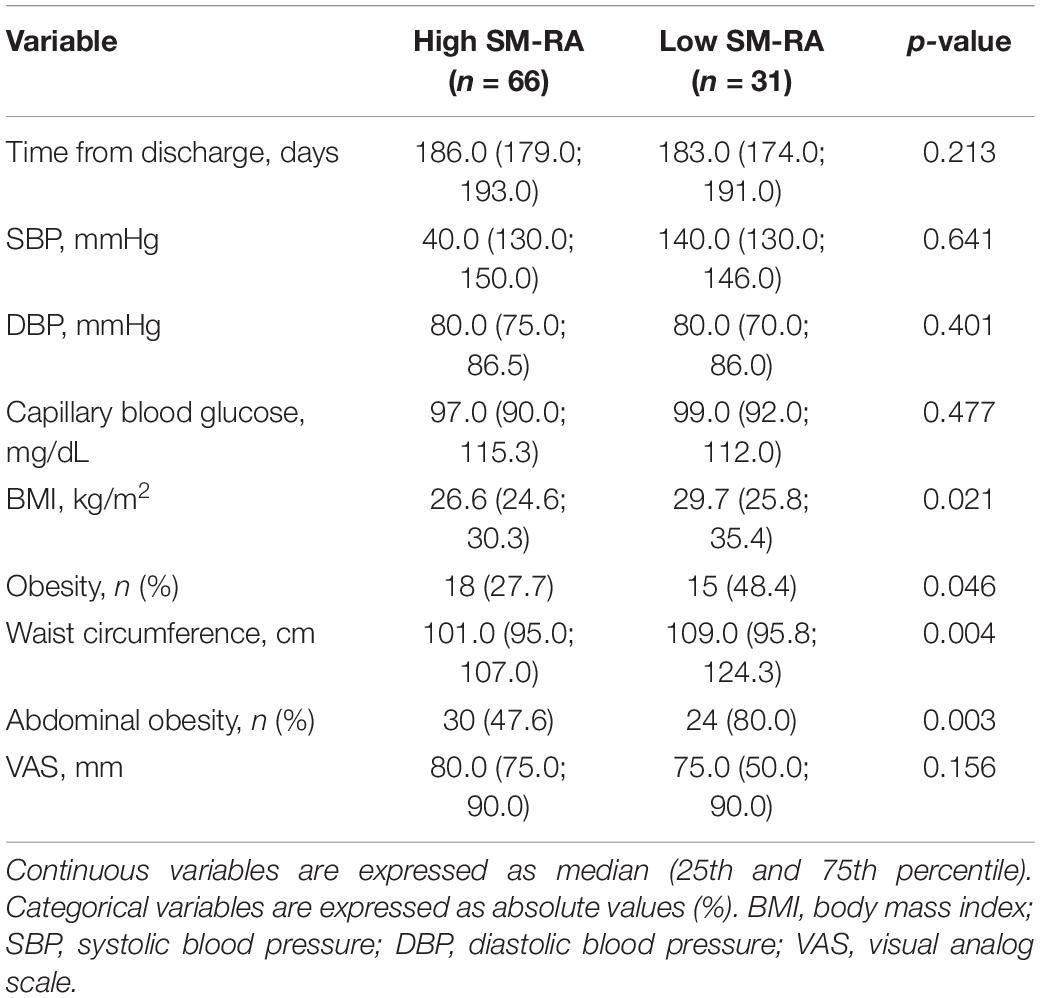
Table 2. Comparison between patients with (low SM-RA) or without (high SM-RA) myosteatosis at 6 months after discharge.
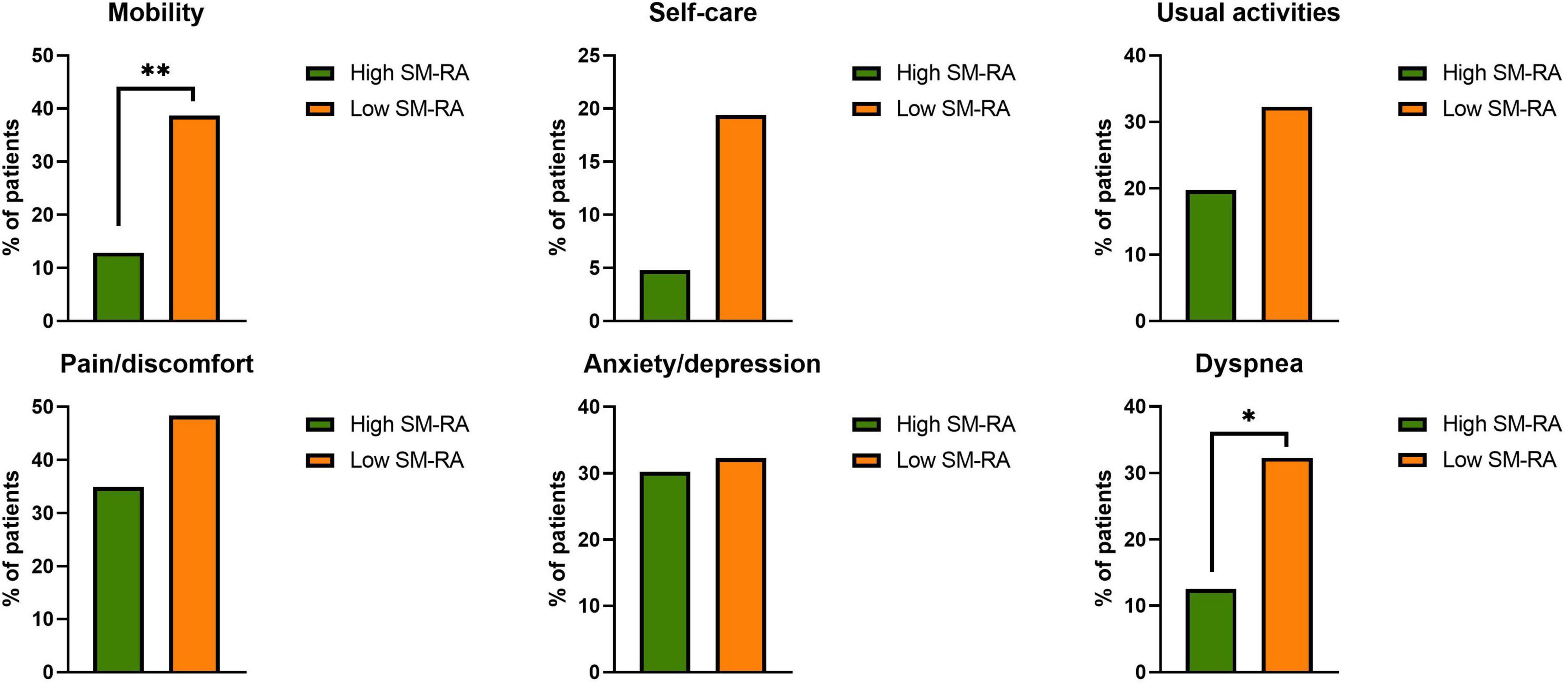
Figure 2. Proportion of patients with any problems in the EQ-5D dimensions (mobility, self-care, usual activities, pain/discomfort, anxiety/depression) and proportion of patients with dyspnea in the group without (high skeletal muscle radiation attenuation, SM-RA) or with (low SM-RA) myosteatosis. *p = 0.021; **p = 0.004.
Effect of Myosteatosis at Hospitalization on Patient-Reported Outcomes at 6 Months After Discharge
To identify predictors of persistent dyspnea at 6 months, we compared anthropometrics and clinical characteristics relative to hospital stay between patients with or without persistent dyspnea. Myosteatosis was significantly more prevalent in those with vs. those without dyspnea (55.6 vs. 27.3%, respectively; p = 0.021), as was obesity (61.1 vs. 28.9%, respectively; p = 0.01). Consistently, patients with persistent dyspnea had significantly lower SM-RA (40.4 [33.6; 47.3] vs. 47.4 [37.7; 51.6] HU in those with vs. those without dyspnea, respectively; p = 0.027), while there was no difference in pSMI (8.9 [7.3; 11.2] vs. 8.9 [7.1; 11.3] in those with vs. those without dyspnea, respectively; p = 0.447). There were no between-group differences in age (60.5 [51.0; 68.0] vs. 60.0 [55.0; 70.5] years in those with vs. those without dyspnea, respectively; p = 0.456), LoS (15.5 [8.0; 42.8] vs. 15.0 [10.0; 28.0] days, p = 0.924) nor in the proportion of males (66.7 vs. 81.8%, p = 0.199), history of arterial hypertension (50.0 vs. 36.8%, p = 0.304), coronary heart disease (16.7 vs. 6.5%, p = 0.172), chronic kidney disease (5.6 vs. 5.2%, p = 1.00), DM (11.1 vs. 10.4%, p = 1.00), or admission to ICU (22.2 vs. 16.9%, p = 0.733). At univariable binomial logistic regression analyses, only myosteatosis was significantly associated with persistent dyspnea at 6 months. Specifically, patients with myosteatosis had threefold the odds to exhibit persistent dyspnea than patients without myosteatosis (OR 3.33 [95% C.I. 1.16; 9.59], p = 0.025). This association remained significant after adjustment for sex, age, and BMI (OR 3.19 [95% C.I. 1.04; 9.87], p = 0.043).
Similarly, myosteatosis was more prevalent in patients with vs. those with no mobility problems (60.0 vs. 26.0%, p = 0.004). Patients with myosteatosis had fourfold the odds to exhibit mobility problems than patients without myosteatosis (OR 4.26 [95% C.I. 1.51; 12.02], p = 0.006. This association remained significant after adjustment for sex, age and BMI (OR 3.70 [95% C.I. 1.25; 10.95], p = 0.018). There were no other significant between-group differences in anthropometrics and clinical characteristics during acute COVID-19.
There was no significant effect of myosteatosis at hospitalization on other EQ-5D dimensions.
Discussion
This is the first analysis exploring the association of myosteatosis with patient-reported outcomes in COVID-19 survivors 6 months after discharge. We showed that myosteatosis, as defined by CT-derived SM-RA during acute COVID-19, significantly predicts persistent dyspnea and mobility problems at 6 months. Our results are consistent with those by van Gassel et al., who reported that myosteatosis, rather than muscle mass, was associated with functional impairment at 3 months after discharge in patients who survived ICU admission for COVID-19 (41). These findings, although novel in the context of COVID-19, are not surprising. In fact, fat infiltration may alter muscle architecture and affect muscle function and strength, increasing the risk of mobility problems (12). In addition, ectopic fat deposition in muscle leads to lipotoxicity, which may contribute to the chronic inflammation associated with obesity (42) and to loss of muscle function and mass (43). Sarcopenia, i.e., reduced muscle function and mass, is associated with reduced pulmonary function in community-dwelling adults (44, 45). Low CT-derived trunk SM-RA is associated with worse physical function and dyspnea in patients with non-small cell lung carcinoma (46), with greater severity of thoracic kyphosis (47), which in turn may impair ventilatory mechanics, and with higher postural sway and worse muscle strength and physical function in community-dwelling adults (24, 48). Although SM-RA may differ depending on the muscle considered (49), the finding that myosteatosis in trunk muscles was associated with impaired physical function in previous studies suggests that SM-RA at this site reflects myosteatosis in other muscles, and is consistent with the association between myosteatosis and mobility problems observed in our cohort.
We found that the prevalence of obesity upon admission was numerically greater in patients with vs. those without myosteatosis (45.2 vs. 25.8%, p = 0.056), this difference reaching statistical significance at the 6-month follow-up (48.4 vs. 27.7%, p = 0.046). Abdominal obesity was also more prevalent among patients with baseline myosteatosis (80.0 vs. 47.6%, p = 0.003). Myosteatosis is strongly associated with obesity, and often precedes the development of type 2 diabetes and the metabolic syndrome (50). Persistent inflammation from both obesity and prior SARS-CoV-2 infection may contribute to the long-term sequelae of COVID-19 (51). Of note, however, the association of myosteatosis with dyspnea and mobility problems was independent of BMI, suggesting that fat infiltration of skeletal muscle per se contributes to these symptoms. Pre-existing myosteatosis may influence long-term outcomes of COVID-19 survivors causing alterations in muscle architecture and lipotoxicity that may impair muscle function. As in a vicious cycle, pre-existing myosteatosis may lead to further worsening of muscle quality. It has been reported that patients with myosteatosis are at increased risk of muscle loss (52). In the setting of COVID-19 this is highly relevant, as acute sarcopenia, i.e., a decline in muscle mass and muscle function within 28 days of a significant physiological stressor event such as an acute illness (53), is emerging as a clinically relevant consequence of SARS-CoV-2 infection (54–56). This may be particularly true in COVID-19 patients more at risk of myosteatosis, i.e., those with overweight or obesity, who exhibit rapid and wide fluctuations in weight that may worsen body composition (57). Factors contributing to muscle wasting in COVID-19 include direct myotoxicity by SARS-CoV-2, systemic inflammation, bed rest, decreased food intake and myotoxic medications (58). Although we did not assess the effect of COVID-19 on muscle quality, we speculate that patients with prior myosteatosis might be more prone to acute sarcopenia, which in turn might contribute to poorer short-term outcomes and slower functional recovery (41, 59–61).
In contrast with other authors who investigated the relationship between opportunistic chest CT-derived SM-RA and short-term outcomes in COVID-19 (28, 62), we did not find an association between myosteatosis in paraspinal muscles and disease severity as reflected by the need for intensive care, which was similar between patients with or without myosteatosis in our cohort. Giraudo et al. have reported that lower CT-derived SM-RA (< 30 HU) predicts ICU admission in COVID-19 patients (62). However, despite patients with lower SM-RA being significantly older, analyses were not adjusted by age. Age (especially > 60 years) has a dramatic effect on COVID-19 severity (63), as well as on myosteatosis, paraspinal muscles being affected more than other muscles (64). It is difficult to argue that myosteatosis increases the risk of ICU based on the analysis by Giraudo et al., the increase in risk being probably due to age differences. The fat content of skeletal muscle is also affected by sex, with women exhibiting lower SM-RA (greater myosteatosis) than men (49, 64, 65). The use of sex- and age-specific cut-offs for defining myosteatosis is a strength of our analysis. Yi et al. found significant associations between measurements of myosteatosis including SM-RA and transition from mild to severe COVID-19 (28). Differences with our findings may be due to study-specific cut-offs for defining myosteatosis, and different patient characteristics (e.g., older age of our cohort). Other authors investigated the relationship between opportunistic chest CT-derived SM-RA and survival using adjustment for several potential confounders including sex and age, reporting significant associations between thoracic myosteatosis and reduced survival in COVID-19 patients (25, 26).
We also calculated pSMI, which is a biomarker of muscle mass (36, 37). In agreement with previous studies showing that CT-derived muscle density is more strongly associated with muscle function than muscle size (24), even in COVID-19 survivors (41), we found that SM-RA, but not pSMI, was associated with dyspnea and mobility problems at 6 months after discharge. This finding highlights the need of assessing muscle quality, in addition to muscle mass.
We must acknowledge some limitations. The study population consisted of patients who visited our COVID-19 outpatient clinic for the 6-month follow-up, which is prone to selection bias and limited generalizability. Direct CT quantification of muscle fat infiltration, which is an additional marker of muscle quality (66), was not available, and patients did not undergo magnetic resonance imaging (MRI) nor measurement of muscle strength for deeper characterization of muscle quality and function. It should be acknowledged, however, that SM-RA is widely recognized as a biomarker of fat infiltration; it is strongly correlated with muscle fat infiltration measured by MRI-derived proton density fat fraction, and is strongly associated with muscle function (14, 67). The lack of a reference measurement and the relatively small sample size of our cohort prevented us from providing SM-RA cut-offs to univocally diagnose myosteatosis in clinical practice. However, the purpose of our analysis was to describe the relationship between myosteatosis and post-discharge outcomes in COVID-19 survivors. Providing cut-off values for the diagnosis of myosteatosis would require a much larger cohort representative of the general population, and the inclusion of healthy subjects.
Conclusion
In conclusion, we showed that myosteatosis detected during acute COVID-19 significantly predicts persistent dyspnea and mobility problems at 6 months after hospital discharge. SM-RA can be directly derived from PACS during imaging reporting, and could be easily applied in clinical practice. Given the potential relevance of opportunistic CT-derived SM-RA in detecting patients at risk of persistent functional impairment after COVID-19, larger studies are needed to identify univocal sex- and age- specific cut-offs for diagnosing myosteatosis and promptly implement interventions aimed at improving muscle health and body composition in COVID-19 survivors, to prevent or minimize the long-term sequelae of the disease.
Data Availability Statement
The raw data supporting the conclusions of this article will be made available by the authors upon reasonable request.
Ethics Statement
The studies involving human participants were reviewed and approved by the Comitato Etico IRCCS Ospedale San Raffaele. The patients/participants provided their written informed consent to participate in this study.
Author Contributions
CC, AE, MM, and PR-Q: conception and design of the work. RD, AP, CG, VN, RL, DV, EF, MF, MC, CM, SM, and GV: acquisition of data. CC and RD: analysis of data. CC, AE, MM, PR-Q, AM, RD, and AP: interpretation of data. All authors have approved the submitted version of the manuscript, and have agreed to be personally accountable for the content of the work.
Funding
Part of this work was supported by a COVID-19 program project grant from the IRCCS San Raffaele Scientific Institute and the grant COVID-2020-12371617 from the Italian Ministero della Salute. CC was supported by the European Foundation for the Study of Diabetes Mentorship Programme 2019.
Conflict of Interest
The authors declare that the research was conducted in the absence of any commercial or financial relationships that could be construed as a potential conflict of interest.
Publisher’s Note
All claims expressed in this article are solely those of the authors and do not necessarily represent those of their affiliated organizations, or those of the publisher, the editors and the reviewers. Any product that may be evaluated in this article, or claim that may be made by its manufacturer, is not guaranteed or endorsed by the publisher.
Acknowledgments
We thank the San Raffaele Scientific Institute Institutional Biobank and the BioB Angels: Nicola Farina, Marco Battista, Domenico Grosso, Francesca Gorgoni, Carlo Di Biase, Alessio Grazioli Moretti, Lucio Granata, Filippo Bonaldi, Giulia Bettinelli, Elena Delmastro, Damiano Salvato, Chiara Maggioni, Giulia Magni, Monica Avino, Paolo Betti, Romina Bucci, Iulia Dumea, Simona Bossolasco, and Federica Morselli.
Supplementary Material
The Supplementary Material for this article can be found online at: https://www.frontiersin.org/articles/10.3389/fnut.2022.846901/full#supplementary-material
References
1. Cuerda C, Sanchez Lopez I, Gil Martinez C, Merino Viveros M, Velasco C, Cevallos Penafiel V, et al. Impact of COVID-19 in nutritional and functional status of survivors admitted in intensive care units during the first outbreak. Preliminary results of the NUTRICOVID study. Clin Nutr. (2021). doi: 10.1016/j.clnu.2021.11.017 [Epub ahead of print].
2. Du HW, Fang SF, Wu SR, Chen XL, Chen JN, Zhang YX, et al. Six-month follow-up of functional status in discharged patients with coronavirus disease 2019. BMC Infect Dis. (2021) 21:1271. doi: 10.1186/s12879-021-06970-3
3. Huang C, Huang L, Wang Y, Li X, Ren L, Gu X, et al. 6-month consequences of COVID-19 in patients discharged from hospital: a cohort study. Lancet. (2021) 397:220–32. doi: 10.1016/S0140-6736(20)32656-8
4. Charfeddine S, Ibn Hadj Amor H, Jdidi J, Torjmen S, Kraiem S, Hammami R, et al. Long COVID 19 syndrome: is it related to microcirculation and endothelial dysfunction? Insights from TUN-EndCOV study. Front Cardiovasc Med. (2021) 8:745758. doi: 10.3389/fcvm.2021.745758
5. Adeloye D, Elneima O, Daines L, Poinasamy K, Quint JK, Walker S, et al. The long-term sequelae of COVID-19: an international consensus on research priorities for patients with pre-existing and new-onset airways disease. Lancet Respir Med. (2021) 9:1467–78. doi: 10.1016/S2213-2600(21)00286-1
6. De Lorenzo R, Conte C, Lanzani C, Benedetti F, Roveri L, Mazza MG, et al. Residual clinical damage after COVID-19: a retrospective and prospective observational cohort study. PLoS One. (2020) 15:e0239570. doi: 10.1371/journal.pone.0239570
7. Fernandez-de-Las-Penas C, Palacios-Cena D, Gomez-Mayordomo V, Palacios-Cena M, Rodriguez-Jimenez J, de-la-Llave-Rincon AI, et al. Fatigue and dyspnoea as main persistent post-COVID-19 symptoms in previously hospitalized patients: related functional limitations and disability. Respiration. (2022) 101:132–141. doi: 10.1159/000518854
8. Fumagalli C, Zocchi C, Tassetti L, Silverii MV, Amato C, Livi L, et al. Factors associated with persistence of symptoms 1 year after COVID-19: a longitudinal, prospective phone-based interview follow-up cohort study. Eur J Intern Med. (2022). 97:36–41. doi: 10.1016/j.ejim.2021.11.018
9. Vimercati L, De Maria L, Quarato M, Caputi A, Gesualdo L, Migliore G, et al. Association between long COVID and overweight/obesity. J Clin Med. (2021) 10:4143. doi: 10.3390/jcm10184143
10. Cox MC, Booth M, Ghita G, Wang Z, Gardner A, Hawkins RB, et al. The impact of sarcopenia and acute muscle mass loss on long-term outcomes in critically ill patients with intra-abdominal sepsis. J Cachexia Sarcopenia Muscle. (2021) 12:1203–13. doi: 10.1002/jcsm.12752
11. Duan K, Gao X, Zhu D. The clinical relevance and mechanism of skeletal muscle wasting. Clin Nutr. (2021) 40:27–37. doi: 10.1016/j.clnu.2020.07.029
12. Naimo MA, Varanoske AN, Hughes JM, Pasiakos SM. Skeletal muscle quality: a biomarker for assessing physical performance capabilities in young populations. Front Physiol. (2021) 12:706699. doi: 10.3389/fphys.2021.706699
13. Ahn H, Kim DW, Ko Y, Ha J, Shin YB, Lee J, et al. Updated systematic review and meta-analysis on diagnostic issues and the prognostic impact of myosteatosis: a new paradigm beyond sarcopenia. Ageing Res Rev. (2021) 70:101398. doi: 10.1016/j.arr.2021.101398
14. Correa-de-Araujo R, Addison O, Miljkovic I, Goodpaster BH, Bergman BC, Clark RV, et al. Myosteatosis in the context of skeletal muscle function deficit: an interdisciplinary workshop at the national institute on aging. Front Physiol. (2020) 11:963. doi: 10.3389/fphys.2020.00963
15. Aubrey J, Esfandiari N, Baracos VE, Buteau FA, Frenette J, Putman CT, et al. Measurement of skeletal muscle radiation attenuation and basis of its biological variation. Acta Physiol (Oxf). (2014) 210:489–97. doi: 10.1111/apha.12224
16. Looijaard WG, Dekker IM, Stapel SN, Girbes AR, Twisk JW, Oudemans-van Straaten HM, et al. Skeletal muscle quality as assessed by CT-derived skeletal muscle density is associated with 6-month mortality in mechanically ventilated critically ill patients. Crit Care. (2016) 20:386. doi: 10.1186/s13054-016-1563-3
17. Boer BC, de Graaff F, Brusse-Keizer M, Bouman DE, Slump CH, Slee-Valentijn M, et al. Skeletal muscle mass and quality as risk factors for postoperative outcome after open colon resection for cancer. Int J Colorectal Dis. (2016) 31:1117–24. doi: 10.1007/s00384-016-2538-1
18. Margadant CC, Bruns ER, Sloothaak DA, van Duijvendijk P, van Raamt AF, van der Zaag HJ, et al. Lower muscle density is associated with major postoperative complications in older patients after surgery for colorectal cancer. Eur J Surg Oncol. (2016) 42:1654–9. doi: 10.1016/j.ejso.2016.05.040
19. Okumura S, Kaido T, Hamaguchi Y, Kobayashi A, Shirai H, Fujimoto Y, et al. Impact of skeletal muscle mass, muscle quality, and visceral adiposity on outcomes following resection of intrahepatic cholangiocarcinoma. Ann Surg Oncol. (2017) 24:1037–45. doi: 10.1245/s10434-016-5668-3
20. Tzeng YH, Wei J, Tsao TP, Lee YT, Lee KC, Liou HR, et al. Computed tomography-determined muscle quality rather than muscle quantity is a better determinant of prolonged hospital length of stay in patients undergoing transcatheter aortic valve implantation. Acad Radiol. (2020) 27:381–8. doi: 10.1016/j.acra.2019.05.007
21. van Dijk DP, Bakens MJ, Coolsen MM, Rensen SS, van Dam RM, Bours MJ, et al. Low skeletal muscle radiation attenuation and visceral adiposity are associated with overall survival and surgical site infections in patients with pancreatic cancer. J Cachexia Sarcopenia Muscle. (2017) 8:317–26. doi: 10.1002/jcsm.12155
22. Hicks GE, Simonsick EM, Harris TB, Newman AB, Weiner DK, Nevitt MA, et al. Cross-sectional associations between trunk muscle composition, back pain, and physical function in the health, aging and body composition study. J Gerontol A Biol Sci Med Sci. (2005) 60:882–7. doi: 10.1093/gerona/60.7.882
23. Jing X, Tan L, Fu H, Yang L, Yang M. Associations of adl disability with trunk muscle mass and muscle quality indicators measured by opportunistic chest computed tomography imaging among older inpatients. Front Med (Lausanne). (2021) 8:743698. doi: 10.3389/fmed.2021.743698
24. Wang L, Yin L, Zhao Y, Su Y, Sun W, Chen S, et al. Muscle density, but not size, correlates well with muscle strength and physical performance. J Am Med Dir Assoc. (2021) 22:751–9.e2. doi: 10.1016/j.jamda.2020.06.052
25. Rossi AP, Gottin L, Donadello K, Schweiger V, Brandimarte P, Zamboni GA, et al. Intermuscular adipose tissue as a risk factor for mortality and muscle injury in critically Ill patients affected by COVID-19. Front Physiol. (2021) 12:651167. doi: 10.3389/fphys.2021.651167
26. Viddeleer AR, Raaphorst J, Min M, Beenen LFM, Scheerder MJ, Vlaar APJ, et al. Intramuscular adipose tissue at level Th12 is associated with survival in COVID-19. J Cachexia Sarcopenia Muscle. (2021) 12:823–7. doi: 10.1002/jcsm.12696
27. Yang Y, Ding L, Zou X, Shen Y, Hu D, Hu X, et al. Visceral adiposity and high intramuscular fat deposition independently predict critical illness in patients with SARS-CoV-2. Obesity (Silver Spring). (2020) 28:2040–8. doi: 10.1002/oby.22971
28. Yi X, Liu H, Zhu L, Wang D, Xie F, Shi L, et al. Myosteatosis predicting risk of transition to severe COVID-19 infection. Clin Nutr. (2021). doi: 10.1016/j.clnu.2021.05.031 [Epub ahead of print].
29. Rovere Querini P, De Lorenzo R, Conte C, Brioni E, Lanzani C, Yacoub MR, et al. Post-COVID-19 follow-up clinic: depicting chronicity of a new disease. Acta Biomed. (2020) 91:22–8. doi: 10.23750/abm.v91i9-S.10146
30. Rovere-Querini P, Tresoldi C, Conte C, Ruggeri A, Ghezzi S, De Lorenzo R, et al. Biobanking for COVID-19 research. Panminerva Med. (2020). doi: 10.23736/S0031-0808.20.04168-3 [Epub ahead of print].
31. von Elm E, Altman DG, Egger M, Pocock SJ, Gotzsche PC, Vandenbroucke JP, et al. The strengthening the reporting of observational studies in epidemiology (STROBE) statement: guidelines for reporting observational studies. J Clin Epidemiol. (2008) 61:344–9. doi: 10.1016/j.jclinepi.2007.11.008
32. Ross R, Neeland IJ, Yamashita S, Shai I, Seidell J, Magni P, et al. Waist circumference as a vital sign in clinical practice: a consensus statement from the IAS and ICCR working group on visceral obesity. Nat Rev Endocrinol. (2020) 16:177–89. doi: 10.1038/s41574-019-0310-7
33. Bestall JC, Paul EA, Garrod R, Garnham R, Jones PW, Wedzicha JA. Usefulness of the medical research council (MRC) dyspnoea scale as a measure of disability in patients with chronic obstructive pulmonary disease. Thorax. (1999) 54:581–6. doi: 10.1136/thx.54.7.581
34. Launois C, Barbe C, Bertin E, Nardi J, Perotin JM, Dury S, et al. The modified medical research council scale for the assessment of dyspnea in daily living in obesity: a pilot study. BMC Pulm Med. (2012) 12:61. doi: 10.1186/1471-2466-12-61
35. EuroQol Research Foundation.EQ-5D. (2021). Available online at: https://euroqol.org/ (accessed December 26, 2021).
36. Amini B, Boyle SP, Boutin RD, Lenchik L. Approaches to assessment of muscle mass and myosteatosis on computed tomography: a systematic review. J Gerontol A Biol Sci Med Sci. (2019) 74:1671–8. doi: 10.1093/gerona/glz034
37. Heymsfield SB, Heo M, Thomas D, Pietrobelli A. Scaling of body composition to height: relevance to height-normalized indexes. Am J Clin Nutr. (2011) 93:736–40. doi: 10.3945/ajcn.110.007161
38. Park J, Gil JR, Shin Y, Won SE, Huh J, You MW, et al. Reliable and robust method for abdominal muscle mass quantification using CT/MRI: an explorative study in healthy subjects. PLoS One. (2019) 14:e0222042. doi: 10.1371/journal.pone.0222042
39. Lee K, Shin Y, Huh J, Sung YS, Lee IS, Yoon KH, et al. Recent issues on body composition imaging for sarcopenia evaluation. Korean J Radiol. (2019) 20:205–17. doi: 10.3348/kjr.2018.0479
40. van der Kroft G, van Dijk DPJ, Rensen SS, Van Tiel FH, de Greef B, West M, et al. Low thoracic muscle radiation attenuation is associated with postoperative pneumonia following partial hepatectomy for colorectal metastasis. HPB (Oxford). (2020) 22:1011–9. doi: 10.1016/j.hpb.2019.10.1532
41. van Gassel RJJ, Bels J, Remij L, van Bussel BCT, Posthuma R, Gietema HA, et al. Functional outcomes and their association with physical performance in mechanically ventilated coronavirus disease 2019 survivors at 3 months following hospital discharge: a cohort study. Crit Care Med. (2021) 49:1726–38. doi: 10.1097/CCM.0000000000005089
42. Miljkovic I, Vella CA, Allison M. Computed tomography-derived myosteatosis and metabolic disorders. Diabetes Metab J. (2021) 45:482–91. doi: 10.4093/dmj.2020.0277
43. Rubio-Ruiz ME, Guarner-Lans V, Perez-Torres I, Soto ME. Mechanisms underlying metabolic syndrome-related sarcopenia and possible therapeutic measures. Int J Mol Sci. (2019) 20:647. doi: 10.3390/ijms20030647
44. Moon JH, Kong MH, Kim HJ. Implication of sarcopenia and sarcopenic obesity on lung function in healthy elderly: using Korean national health and nutrition examination survey. J Korean Med Sci. (2015) 30:1682–8. doi: 10.3346/jkms.2015.30.11.1682
45. Ohara DG, Pegorari MS, Oliveira Dos Santos NL, de Fatima Ribeiro Silva C, Oliveira MSR, Matos AP, et al. Cross-sectional study on the association between pulmonary function and sarcopenia in brazilian community-dwelling elderly from the amazon region. J Nutr Health Aging. (2020) 24:181–7. doi: 10.1007/s12603-019-1290-y
46. Bye A, Sjoblom B, Wentzel-Larsen T, Gronberg BH, Baracos VE, Hjermstad MJ, et al. Muscle mass and association to quality of life in non-small cell lung cancer patients. J Cachexia Sarcopenia Muscle. (2017) 8:759–67. doi: 10.1002/jcsm.12206
47. Lorbergs AL, Allaire BT, Yang L, Kiel DP, Cupples LA, Jarraya M, et al. A longitudinal study of trunk muscle properties and severity of thoracic kyphosis in women and men: the framingham study. J Gerontol A Biol Sci Med Sci. (2019) 74:420–7. doi: 10.1093/gerona/gly056
48. Anderson DE, Quinn E, Parker E, Allaire BT, Muir JW, Rubin CT, et al. Associations of computed tomography-based trunk muscle size and density with balance and falls in older adults. J Gerontol A Biol Sci Med Sci. (2016) 71:811–6. doi: 10.1093/gerona/glv185
49. Figueiredo P, Marques EA, Gudnason V, Lang T, Sigurdsson S, Jonsson PV, et al. Computed tomography-based skeletal muscle and adipose tissue attenuation: variations by age, sex, and muscle. Exp Gerontol. (2021) 149:111306. doi: 10.1016/j.exger.2021.111306
50. Hausman GJ, Basu U, Du M, Fernyhough-Culver M, Dodson MV. Intermuscular and intramuscular adipose tissues: Bad vs. good adipose tissues. Adipocyte. (2014) 3:242–55. doi: 10.4161/adip.28546
51. Khwatenge CN, Pate M, Miller LC, Sang Y. Immunometabolic dysregulation at the intersection of obesity and COVID-19. Front Immunol. (2021) 12:732913. doi: 10.3389/fimmu.2021.732913
52. Tachi Y, Kozuka A, Hirai T, Ishizu Y, Honda T, Kuzuya T, et al. Impact of myosteatosis on skeletal muscle volume loss in patients with chronic liver disease. J Gastroenterol Hepatol. (2018). doi: 10.1111/jgh.14133 [Epub ahead of print].
53. Welch C, K Hassan-Smith Z, A Greig C, M Lord J, A Jackson T. Acute sarcopenia secondary to hospitalisation – an emerging condition affecting older adults. Aging Dis. (2018) 9:151–64. doi: 10.14336/AD.2017.0315
54. Morley JE, Kalantar-Zadeh K, Anker SD. COVID-19: a major cause of cachexia and sarcopenia? J Cachexia Sarcopenia Muscle. (2020) 11:863–5. doi: 10.1002/jcsm.12589
55. Piotrowicz K, Gasowski J, Michel JP, Veronese N. Post-COVID-19 acute sarcopenia: physiopathology and management. Aging Clin Exp Res. (2021) 33:2887–98. doi: 10.1007/s40520-021-01942-8
56. Welch C, Greig C, Masud T, Wilson D, Jackson TA. COVID-19 and acute sarcopenia. Aging Dis. (2020) 11:1345–51. doi: 10.14336/AD.2020.1014
57. Di Filippo L, De Lorenzo R, Cinel E, Falbo E, Ferrante M, Cilla M, et al. Weight trajectories and abdominal adiposity in COVID-19 survivors with overweight/obesity. Int J Obes (Lond). (2021) 45:1986–94. doi: 10.1038/s41366-021-00861-y
58. Ali AM, Kunugi H. Skeletal muscle damage in COVID-19: a call for action. Medicina (Kaunas). (2021) 57:372. doi: 10.3390/medicina57040372
59. Damanti S, Cristel G, Ramirez GA, Bozzolo EP, Da Prat V, Gobbi A, et al. Influence of reduced muscle mass and quality on ventilator weaning and complications during intensive care unit stay in COVID-19 patients. Clin Nutr. (2021). doi: 10.1016/j.clnu.2021.08.004 [Epub ahead of print].
60. Gil S, Jacob Filho W, Shinjo SK, Ferriolli E, Busse AL, Avelino-Silva TJ, et al. Muscle strength and muscle mass as predictors of hospital length of stay in patients with moderate to severe COVID-19: a prospective observational study. J Cachexia Sarcopenia Muscle. (2021) 12:1871–8. doi: 10.1002/jcsm.12789
61. Molfino A, Imbimbo G, Rizzo V, Muscaritoli M, Alampi D. The link between nutritional status and outcomes in COVID-19 patients in ICU: is obesity or sarcopenia the real problem? Eur J Intern Med. (2021) 91:93–5. doi: 10.1016/j.ejim.2021.06.028
62. Giraudo C, Librizzi G, Fichera G, Motta R, Balestro E, Calabrese F, et al. Reduced muscle mass as predictor of intensive care unit hospitalization in COVID-19 patients. PLoS One. (2021) 16:e0253433. doi: 10.1371/journal.pone.0253433
63. Bonanad C, Garcia-Blas S, Tarazona-Santabalbina F, Sanchis J, Bertomeu-Gonzalez V, Facila L, et al. The effect of age on mortality in patients with COVID-19: a meta-analysis with 611,583 subjects. J Am Med Dir Assoc. (2020) 21:915–8. doi: 10.1016/j.jamda.2020.05.045
64. Anderson DE, D’Agostino JM, Bruno AG, Demissie S, Kiel DP, Bouxsein ML. Variations of CT-based trunk muscle attenuation by age, sex, and specific muscle. J Gerontol A Biol Sci Med Sci. (2013) 68:317–23. doi: 10.1093/gerona/gls168
65. Schafer AL, Vittinghoff E, Lang TF, Sellmeyer DE, Harris TB, Kanaya AM, et al. Fat infiltration of muscle, diabetes, and clinical fracture risk in older adults. J Clin Endocrinol Metab. (2010) 95:E368–72. doi: 10.1210/jc.2010-0780
66. Tan L, Ji G, Bao T, Fu H, Yang L, Yang M. Diagnosing sarcopenia and myosteatosis based on chest computed tomography images in healthy Chinese adults. Insights Imaging. (2021) 12:163. doi: 10.1186/s13244-021-01106-2
Keywords: myosteatosis, SARS-CoV-2, sarcopenia, long-COVID, obesity, skeletal muscle, dyspnea
Citation: De Lorenzo R, Palmisano A, Esposito A, Gnasso C, Nicoletti V, Leone R, Vignale D, Falbo E, Ferrante M, Cilla M, Magnaghi C, Martinenghi S, Vitali G, Molfino A, Rovere-Querini P, Muscaritoli M and Conte C (2022) Myosteatosis Significantly Predicts Persistent Dyspnea and Mobility Problems in COVID-19 Survivors. Front. Nutr. 9:846901. doi: 10.3389/fnut.2022.846901
Received: 31 December 2021; Accepted: 10 March 2022;
Published: 08 April 2022.
Edited by:
Alice Sabatino, University of Parma, ItalyReviewed by:
Giuseppe Regolisti, University of Parma, ItalySusan Tsivitse Arthur, University of North Carolina at Charlotte, United States
Copyright © 2022 De Lorenzo, Palmisano, Esposito, Gnasso, Nicoletti, Leone, Vignale, Falbo, Ferrante, Cilla, Magnaghi, Martinenghi, Vitali, Molfino, Rovere-Querini, Muscaritoli and Conte. This is an open-access article distributed under the terms of the Creative Commons Attribution License (CC BY). The use, distribution or reproduction in other forums is permitted, provided the original author(s) and the copyright owner(s) are credited and that the original publication in this journal is cited, in accordance with accepted academic practice. No use, distribution or reproduction is permitted which does not comply with these terms.
*Correspondence: Caterina Conte, caterina.conte@uniroma5.it
†These authors have contributed equally to this work and share first authorship
‡These authors have contributed equally to this work and share last authorship