- 1Infectivology and Clinical Trials Research Department, Children’s Hospital Bambino Gesù, Rome, Italy
- 2Department of Clinical and Molecular Medicine, Azienda Ospedaliera-Universitaria S. Andrea, Sapienza University, Rome, Italy
- 3Research Laboratories, Children’s Hospital Bambino Gesù, Rome, Italy
- 4Department of Clinical Internal Sciences, Anesthesiology and Cardiovascular Sciences, Sapienza University of Rome, Rome, Italy
- 5Unit of Endocrine Pathology of Post-Tumoral and Chronic Diseases, Children’s Hospital Bambino Gesù, Rome, Italy
- 6Institute of Cell Biology and Neurobiology, National Research Council of Italy (CNR), Rome, Italy
Carcinomas evade the host immune system by negatively modulating CD4+ and CD8+ T effector lymphocytes through forkhead box protein 3 (FOXP3) positive T regulatory cells’ increased activity. Furthermore, interaction of the programmed cell death 1 (PD1) molecule and its ligand programmed cell death ligand 1 (PDL1) inhibits the antitumor activity of PD1+ T lymphocytes. Immunotherapy has become a powerful strategy for tailored cancer patients’ treatment both in adult and pediatric patients aiming to generate potent antitumor responses. Nevertheless, immunotherapies can generate autoimmune responses. This study aimed to investigate the potential effect of the transformation-related protein 53 (p53) reactivation by a peptide-based inhibitor of the MDM2/MDM4 heterodimer (Pep3) on the immune response in a solid cancer, i.e., thyroid carcinoma frequently presenting with thyroid autoimmunity. In peripheral blood mononuclear cell of thyroid cancer patients, Pep3 treatment alters percentages of CD8+ and CD4+ T regulatory and CD8+ and CD4+ T effector cells and favors an anticancer immune response. Of note that reduced frequencies of activated CD8+ and CD4+ T effector cells do not support autoimmunity progression. In evaluating PD1 expression under p53 activation, a significant decrease of activated CD4+PD1+ cells was detected in thyroid cancer patients, suggesting a defective regulation in the initial activation stage, therefore generating a protective condition toward autoimmune progression.
Introduction
Tumor cells have the ability to evade the host immune system (1, 2). Immune escaping is a common feature of carcinomas and is achieved by multiple mechanisms, one of which is the negative modulation of CD4+ and CD8+ T effector (Teff) lymphocytes, caused by the activity of forkhead box protein 3 (FOXP3)-positive T regulatory cells (Treg) (3). Another mechanism occurs upon the interaction of the programmed cell death 1 (PD1) molecule and its ligand programmed cell death ligand 1 (PDL1), often expressed by tumor cells, which inhibits the antitumor activity of PD1+ Teff lymphocytes (4).
Remarkably, PDL1 overexpression has been detected in the microenvironment of different cancer conditions including mammary and colon adenocarcinomas (5), and PD1 is upregulated in tumor-infiltrating Treg and Teff cells, contributing to Teff exhaustion in tumor lymphocyte infiltration (1, 6). PD1 expression on CD8+ Teff and Treg indeed affects tumor regression, acting on their activity against tumor cells or immunosuppression, respectively (7). Furthermore, additional immune-suppressive receptors are upregulated in the cancer inflammatory microenvironment, i.e., T cell immunoglobulin mucin 3 (TIM-3), cytotoxic T lymphocytes antigen 4 (CTLA-4), glucocorticoid-induced TNFR (tumor necrosis factor receptor)-related protein (GITR), and lymphocyte-activation gene 3 (LAG-3) (8). On a speculative basis, one can envisage that the overexpression of these molecules correlates with the higher suppressive activity of tumor-infiltrating Treg (8).
In light of the foregoing, immunotherapy has become a powerful clinical strategy for tailored treatment of cancer patients. Several approaches have been implemented to control the modulation of the immune system and generate potent antitumor immune responses with fewer side effects compared to standard chemotherapies and other drugs that directly kill cancer cells (9). Immunotherapies restore different physiological mechanisms, which are evaded during cancer progression, leading to the activation or boost of effector cells against cancer cells. Nevertheless, immunotherapies can face several challenges in terms of both efficacy and safety since these treatments can generate autoimmune responses in some patients with the destruction of healthy tissues (10). Regarding immunotherapy delivery systems, these include checkpoint inhibitors, i.e., anti-PD1 (pembrolizumab, nivolumab, cemiplimab), anti-PDL1 (atezolizumab, avelumab, durvalumab), anti-PD1/PDL1 (AUNP12, under experimentation), and anti-CTLA-4 (ipilimumab) monoclonal antibodies, cytokines promoting lymphocyte activation, chimeric antigen receptor (CAR) and T cell receptor (TCR) engineered T cells, agonist antibodies against co-stimulatory receptor and cancer vaccines (9). In particular, PD1-PDL1 blocking antibodies can enhance the activity of tumor-specific Teff cells, modulate Treg function, and reduce anti-inflammatory interleukin (IL-10) while enhancing proinflammatory cytokines release (10). Interestingly, in the tumor microenvironment, the balance of PD1+ CD8+ Teff and Treg lymphocytes is a putative marker of the clinical response to PD1 blockade more reliable than expression of PDL1 or cancer mutation burden (7). Combined impairment of CTLA-4 and PD1 signal transduction showed efficacy especially in some patients affected by melanoma (11, 12). Sole inhibition of CTLA-4 has been recommended for the risk of toxicity and autoimmune manifestations, while PD1 blockage led to milder immunity against self [reviewed (rev) in (1)].
On exploring future avenues for cancer treatment especially aimed at ameliorating prognosis overall in the pediatric population, we envisage the potential use of p53 (transformation-related protein 53) reactivation (13, 14). p53 is a short-lived transcription factor part of a sequence-specific family including p63 and p73 (14). The activity of p53 in normal cells is under the control of two primary regulators, MDM2 (mouse double minute 2) and MDM4 (mouse double minute 4) proteins. MDM2 is a ubiquitin ligase able to bind p53. The subsequent p53 ubiquitination leads to its proteasome-dependent degradation. MDM2 activity is enhanced when it forms a heterodimer with MDM4 (15, 16). When p53 is detached from MDM4 and MDM2 or the heterodimer is dissociated, activation of the protein occurs (17). The protein, expressed at low concentration in various tissues, is found at high levels in damaged, tumoral, or inflamed conditions, playing a relevant regulatory function on gene expression [rev in (13, 17, 18)]. In human cancers, p53 mediates a crucial tumor suppression activity [rev in (13, 14)], by affecting different functional pathways: arrest cell cycle progression, program cell death including apoptosis and autophagy; induce senescence; alter metabolism, fertility, and stem cell development and regulation [rev in (13)]. p53 has also immune regulatory functions (19) by suppressing inflammation and autoimmunity in animal models and in humans (3, 19–21). In patients affected by rheumatoid arthritis (RA), which have lower p53 mRNA levels and higher percentages of circulating T helper 17 (Th17) cells compared to healthy controls, the inhibition of p53-MDM2 interaction by Nutlin-3a induced Treg differentiation under the effect of Th17 polarizing conditions (3). In Type 1 diabetes (T1D) patients, we proved that p53 activation increases the percentages of CD8+ Treg; however, CD8+ T cell subset was also increased, especially the frequency of activated Teff cells that may negatively influence disease progression (21). This further envisages that p53 activation immunotherapy cannot be administered to all categories of autoimmune disease patients or could be beneficial especially in conditions in which patients present a defect of wild-type p53 expression (3).
In this study, we aimed at investigating the consequences of p53 reactivation by Pep3, a peptide inhibitor of the MDM2/MDM4 heterodimerization (14), on the immune response in a model of solid cancer, i.e., thyroid carcinoma that frequently occurs in association with thyroid autoimmunity (22, 23). The investigation was conducted to gain new insights and open immunotherapeutic perspectives to manage this condition both in adult and pediatric patients.
Materials and Methods
Subjects
Eleven patients affected by thyroid carcinoma (TC) were analyzed (Table 1). Within the group of TC patients recruited in the present study, the mean age was 51 years (ranging from 30 to 62 years, one male, 10 females). Subjects were enrolled in the follow-up for thyroid carcinoma with a mean of 9.7 years (yrs) of disease post-surgery (ranging from 6 months to 28 yrs) at the Department of Endocrinology, Azienda Ospedaliera-Universitaria S. Andrea, Sapienza University, Rome over the past 28 yrs. Sera from patients were analyzed for the presence of thyroglobulin (Tg) and thyroperoxidase (TPO) autoantibodies (AAbs) tested by chemiluminescence (ADVIA Centaur analyzer: Siemens Healthcare, Germany). All controls were matched to patients for sex, age, and ethnic and geographical origin. Recruited patients and controls were unrelated. The local Institutional Review Boards (IRB) of the Bambino Gesù Children’s Hospital (OPBG) and Sapienza University have approved the study, and all participants gave written informed consent in accordance with the Declaration of Helsinki.
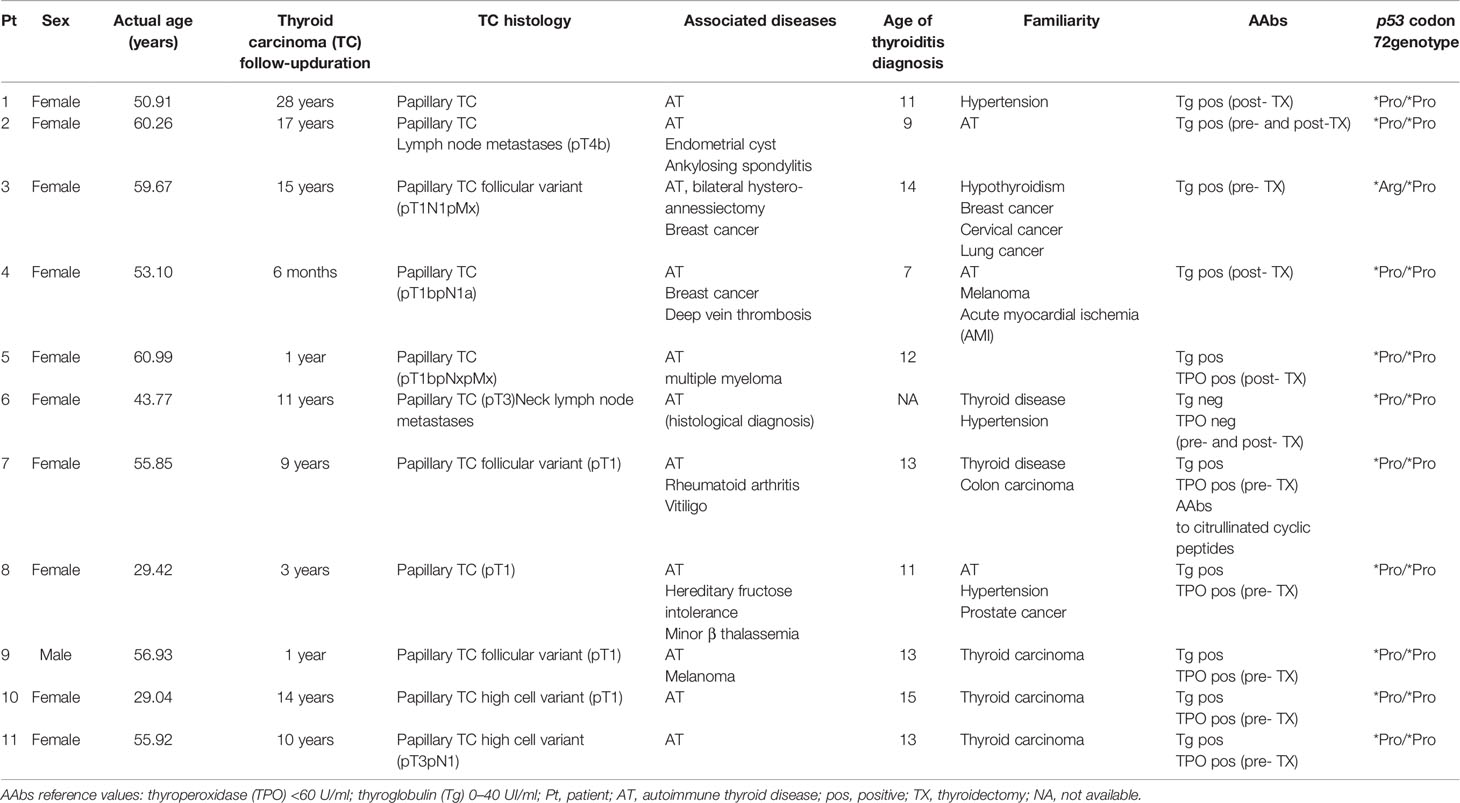
Table 1 Demographic, clinical characteristics, and thyroid autoantibody levels in patients with thyroid carcinoma recruited for the study.
Detection of p53 Codon 72 Polymorphism in Patients With Thyroid Cancer
Genomic leukocyte DNA was extracted from whole blood samples of patients by QIAmp DNA blood mini kit (Qiagen, Hilden Germany) according to the manufacturer’s guidelines. Polymerase chain reaction (PCR) was carried out with specific primers for exon 4 of the gene (Gene Bank ID:7157): forward 5’-AATGGATGATTGATGCTGTCCC-3’ and reverse 5’ GGTGCAAGTCACAGACTTGGC-3’ (24). The amplification lasted 35 cycles with 62°C annealing temperature. PCR sequencing was carried out with the BigDye Terminator v.3.1 Cycle sequencing protocol (Life Technologies, Applied Biosystems, Paisley, Scotland, UK). Products were then purified and sequenced with the Genetic Analyzer 3500 (Applied Biosystems HITACHI system).
Cell Preparation and Culture Conditions
Peripheral blood mononuclear cells (PBMC) from TC patients and healthy donors (HD) were isolated from sodium heparinized venous blood samples (10 ml) using Ficoll-Hypaque (Histopaque, Sigma-Aldrich Chemical Company, St. Louis, MO, USA) and then frozen in liquid nitrogen according to standard protocols (25, 26). Liquid-nitrogen frozen TC and HD PBMC were quickly thawed in pre-warm RPMI medium (GibcoTM RPMI 1640 Medium, ThermoFisher Scientific, Waltham, MA, USA) supplemented with 10% fetal bovine serum (FBS, Hyclone, South Logan, UT, USA), L-glutamine (2 mM) (EuroClone S.p.A, Milan, Italy), penicillin (100 U/ml) (EuroClone S.p.A, Milan, Italy), and streptomycin (100 μg/ml) (EuroClone S.p.A, Milan, Italy) according to established protocols (26). Cells were centrifuged at 1,200 rpm for 5 min at room temperature (RT) and seeded into 48-well plates (Falcon, Corning 7 Incorporated, NY, USA) at density of 1.5×106 cells per well in a final volume of 250 µl. Subsequently, the cells were treated with different doses of peptide 3 (Pep3) (10, 15, and 20 µM) or peptide 3 mutated (Pep3 MUT) (15 µM) as previously described (14). As reported in the literature, Pep3 is composed of 12 aminoacids and selectively impairs the MDM2/MDM4 heterodimer formation, thus activating the p53 function (14). Pep3 MUT has the same sequence of Pep3, except for a mutation in the key contact point of the peptide. The mutation, in turn, makes this peptide unable to impair the heterodimer formation; therefore, Pep3 MUT represents Pep3 specificity control for the experiment.
After 24 hours (h) of incubation, the cells were subsequently stimulated with the addition of Dynabeads Human T-activator CD3/CD28 (Life Technologies AS, Oslo, Norway) at a bead-to-cell ratio of 1:50, reflecting the physiological conditions and allowing the detection of immunomodulatory activity (25). The cells were harvested at 4 and 6 days after stimulation with anti-CD3/CD28 beads, and they were washed by centrifugation at 1,200 rpm for 5 min RT in phosphate-buffered saline (PBS, EuroClone S.p.A, Milan, Italy) before being used for flow cytometry analysis (FACS).
Flow Cytometry Analysis
In order to analyze T-cell subsets, cells were stained as already described (27) for 20 min at 4°C for surface markers detection. The antibodies used are listed as follows: Brilliant Ultraviolet 737 (BUV737) conjugated mouse anti-human CD3 (Clone UCHT1; 1:40 dilution; BD Biosciences, CA, USA); Brilliant Violet 421 (BV421) conjugated mouse anti-human CD25 (Clone M-A251; 1:40 dilution; BD); allophycocyanin (APC) conjugated mouse anti-human CD8 (Clone RPA-T8; 1:10 dilution; BD); and R-phycoerythrin-Cyanine7 (PE-Cy7) conjugated mouse anti-human CD279 (programmed cell death 1, PD1) (Clone J105; 1:40 dilution; eBioscience, ThermoFisher Scientific). After incubation, cells were washed once with wash buffer (2% FBS in PBS) and centrifuged at 1,200 rpm for 5 min at RT. Subsequently, cells underwent FOXP3 intracellular staining using PE conjugated mouse anti-human FOXP3 antibody (Clone 259D/C7, BD) following the manufacturer’s protocol (Human FOXP3 Buffer Set, BD). According to literature, CD8+CD25+FOXP3+ cells were considered as CD8+ Treg and CD8+CD25−FOXP3− cells as CD8+ effector T cells (Teff) (28, 29). CD8+CD25+FOXP3− cells were considered activated CD8+ Teff cells. PD1+ cells within the total gate of CD8+ Treg and Teff were identified as CD8+ Treg PD1+ cells and CD8+Teff PD1+ cells. Moreover, in this study the same populations as described above were also evaluated among the CD4+ subset (Figure S1). Data were acquired using a Fortessa X-20 flow-cytometer [Becton and Dickinson (BD), Sunnyvale, CA, USA] and analyzed by FACSDiva software (BD Biosciences: San Jose, CA, USA). Dead cells were excluded from the analysis by side/forward scatter gating (Figure S1), and 50,000 events/sample were acquired on the lymphocyte gate.
Statistical Analysis
Data are expressed as mean ± SEM. Statistical significance was evaluated among the treatments for all subsets analyzed in 11 TC patients by Wilcoxon matched-pairs signed rank test. Differences between HD and TC among the different treatments in terms of percentages of PD1+ cells among subsets analyzed were assessed by the Mann Whitney test. The analysis was carried out with the GraphPad Prism software version number 7.00 (GraphPad Software: San Diego, CA, USA). A result of p < 0.05 was considered statistically significant.
Results
Study Population
Demographic, clinical, and laboratory characteristics of patients are shown in Table 1. All subjects enrolled in the study are of Caucasian origin, coming from Central Italy. In addition to thyroid carcinoma, 10 patients (over 90% of total patients) also developed autoimmune thyroiditis (AT) and one patient (Pt) had AT histological diagnosis (Pt 6, Table 1). Among other autoimmune disorders, one patient was affected by ankylosing spondylitis (Pt 2, Table 1) and one patient by rheumatoid arthritis and vitiligo (Pt 7, Table 1). Associated cancer conditions included breast cancer (Pts 3–4, Table 1), multiple myeloma (Pt 5, Table 1), and melanoma (Pt 9, Table 1). Nine patients had family history for thyroid disorders; three patients (Pts 9–11, Table 1) had family history for thyroid cancer; one patient for breast cancer (Pt 3, Table 1), one patient for cervical cancer (Pt 3, Table 1), and one patient for colon cancer (Pt 7, Table 1). All patients are characterized by papillary thyroid cancer, a histotype associated with wt-p53 in almost 100% of cases (30). Since wtp53 with a polymorphism at codon 72 (p53P72) is associated with an increased risk of cancer due to the decreased ability of p53P72 to induce apoptosis (31), the polymorphism characterization was evaluated in the patients cohort of this study. Indeed, p53 *Arg/*Pro is present in one patient (Pt 3, Table 1), and the remaining 10 harbor the *Pro/*Pro genotype. Patients were recruited during the follow-up of thyroid carcinoma, which was diagnosed in adult age except for Pt 10, Table 1.
Peptide 3 Efficacy on Thyroid Cancer PBMC Subsets
In order to evaluate the effect of Pep3 in TC PBMC patients, different doses of Pep3, which been previously demonstrated efficacious (14), were used. In TC PBMC pretreated with Pep3 and subsequently stimulated for 4 days with anti-CD3/CD28 beads, the percentage of CD8+ Treg (CD8+CD25+FOXP3+) cells significantly decreased at the lowest dose used (10 μM) and did not further decrease at higher doses (15 and 20 μM) compared to untreated stimulated cells (Figures 1A and S2). As expected, the control peptide, Pep3 MUT, did not alter the frequency of CD8+ Treg cells, indicating the specific activity of Pep3. Conversely, the percentage of CD8+ Teff (CD8+CD25−FOXP3−) cells increased significantly upon Pep3 treatment (Figures 1B and S2); as a consequence, the ratio between the two subsets decreased (Figure 1C). Notably, the CD8+ Teff activated cell subset (CD8+CD25+FOXP3−) decreased significantly upon stimulation with anti-CD3/CD28 beads following pretreatment with the Pep3 at the same concentrations (Figures 1D and S2), meaning increased effect at 10 and 15 μM Pep3 treatment (Figure 1D). Again, no significant change was detected in cultures pretreated with Pep3 MUT at the dose of 15 μM, confirming the specificity of Pep3 activity (Figures 1B–D). Regarding CD4+ Treg (CD4+CD25+FOXP3+) cells, under the same conditions, percentages were decreased after Pep3 treatment (Figure 1E), while a significant increase was detected in CD4+ Teff (CD4+CD25−FOXP3−) cells (Figure 1F). Consequently, the CD4+ Treg/Teff ratio decreased after Pep3 treatment (Figure 1G). As observed above, CD4+ Teff activated cells (CD4+CD25+FOXP3−) significantly decreased in percentage and in a dose-dependent manner from 10 to 15 μM of Pep3 treatment (Figure 1H). These results indicate that in PBMC of patients affected by thyroid cancer, Pep3 treatment can alter the percentages of CD8+ and CD4+ Treg, CD8+ and CD4+ Teff cells, as well as of CD8+ and CD4+ Teff activated cells and favor an anticancer immune response. Importantly, the reduced frequencies of activated CD8+ and CD4+ Teff cells observed, in vitro, in PBMC of patients affected by thyroid cancer associated with autoimmune thyroiditis at time of analysis, do not foresee progression of autoimmune responses post-thyroidectomy in vivo during the follow-up.
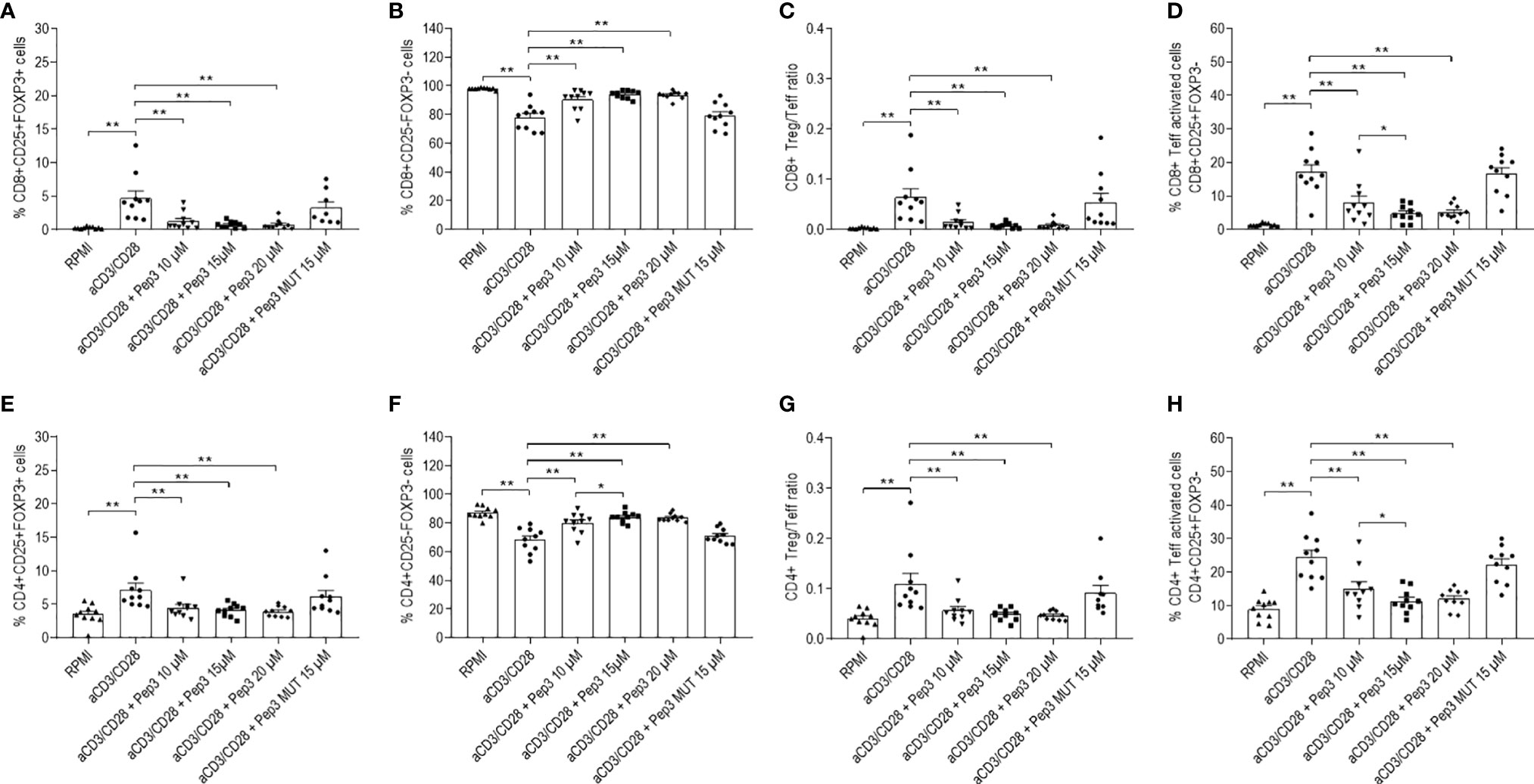
Figure 1 Frequency of T-cell populations in PBMC isolated from TC patients treated with peptide 3 and subsequently stimulated with anti-CD3/CD28 beads for 4 days. Flow-cytometry analysis of TC PBMC untreated unstimulated (RPMI) or stimulated (anti-CD3/CD28), treated with 10, 15, and 20 µM Pep3 or 15 µM of Pep3 MUT and subsequently stimulated with anti-CD3/CD28 beads for 4 days. Percentages of CD8+ subsets (CD8+ Treg, CD8+ Teff, CD8+ Teff activated) were calculated on total CD8+ cells. Percentages of CD4+ subsets (CD4+ Treg, CD4+ Teff, CD4+ Teff activated) were calculated on total CD4+ cells. Percentages of total CD8+ and CD4+ cells were calculated on total lymphocytes. Graphs show the percentages upon CD3/CD28 stimulation of CD8+ Treg as CD8+CD25+FOXP3+ cells (A), CD8+ Teff as CD8+CD25−FOXP3− cells (B), CD8+ Treg/Teff ratio (C), CD8+ Teff activated cells as CD8+CD25+FOXP3− cells (D), CD4+ Treg as CD4+CD25+FOXP3+ cells (E), CD4+ Teff as CD4+CD25−FOXP3− cells (F) (please refer for gating strategy to S1 Figure), CD4+ Treg/Teff ratio (G), percentage of CD4+ Teff activated cells as CD4+CD25+FOXP3− cells (H). Statistical significance was evaluated using Wilcoxon matched-pairs signed rank test. Data are expressed as mean ± SEM, and each dot corresponds to an individual TC patient (n = 10). *p < 0.05, **p < 0.01.
Effects of Pep3 exposure were still persistent after 6 days of stimulation with anti-CD3/CD28 beads, indicating the long-term activity of Pep3 on p53 activation (Figures 2A–H). Particularly, the effects on CD8+ Treg lowered but were still significant as at 4 days (Figure 2A). Effects on CD8+ Teff, CD4+ Teff (Figures 2B, F) and on activated CD8+ and CD4+ Teff (Figures 2D, H) cells were even higher compared to 4 days of treatment (Figure S3). Conversely, no effect was observed on CD4+ Treg (Figure 2E). As expected from these results, after 6 days, the relative ratios were substantially altered by Pep3 treatment (Figure 2G).
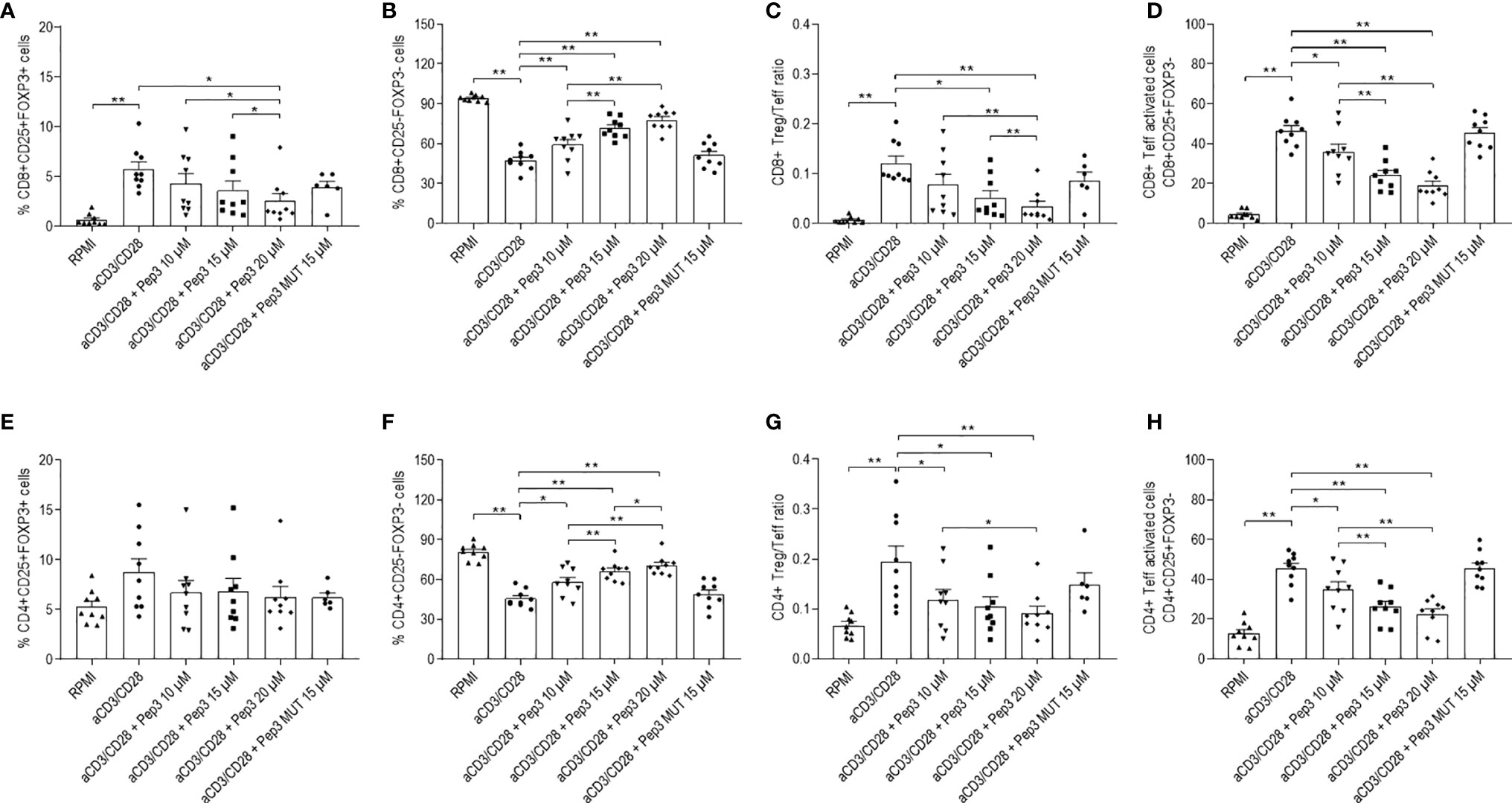
Figure 2 Frequency of T cell populations in PBMC from TC patients upon treatment with peptide 3 and subsequent stimulation with anti-CD3/CD28 beads for 6 days. Flow-cytometry analysis of TC PBMC following 10, 15, and 20 µM treatment relative to Pep3 and 15 µM treatment relative to Pep3 MUT and subsequent stimulation for 6 days with anti-CD3/CD28 beads. Graphs show the percentages upon CD3/CD28 stimulation of CD8+ Treg as CD8+CD25+FOXP3+ cells (A), CD8+ Teff as CD8+CD25−FOXP3− cells (B), CD8+ Treg/Teff ratio (C), CD8+ Teff activated cells as CD8+CD25+FOXP3− cells (D), CD4+ Treg as CD4+CD25+FOXP3+ cells (E), CD4+ Teff as CD4+CD25−FOXP3− cells (F), CD4+ Treg/Teff ratio (G), CD4+ Teff activated cells as CD4+ CD25+FOXP3- cells (H). Data are expressed as mean ± SEM, and each dot corresponds to an individual TC patient (n = 9). Statistical significance was evaluated using Wilcoxon matched-pairs signed rank test. *p < 0.05, **p < 0.01.
Peptide 3 Efficacy on the Expression of PD1 Molecule in Thyroid Cancer PBMC Subsets
With the purpose of investigating T cell activation following Pep3 reactivation, expression of the regulatory PD1 molecule was studied after 24 h of Pep3 treatment and subsequent anti-CD3/CD28 stimulation (Figure 3A–F, 4A–F).
After 4 days of stimulation, Pep3 administration increased percentages of CD8+ Treg PD1+ cells (Figure 3A) and CD8+ Teff activated PD1+ (Figure 3C) at 20 and 10 µM, respectively, where no such effects were observed in CD8+ Teff PD1+ cells (Figure 3B).
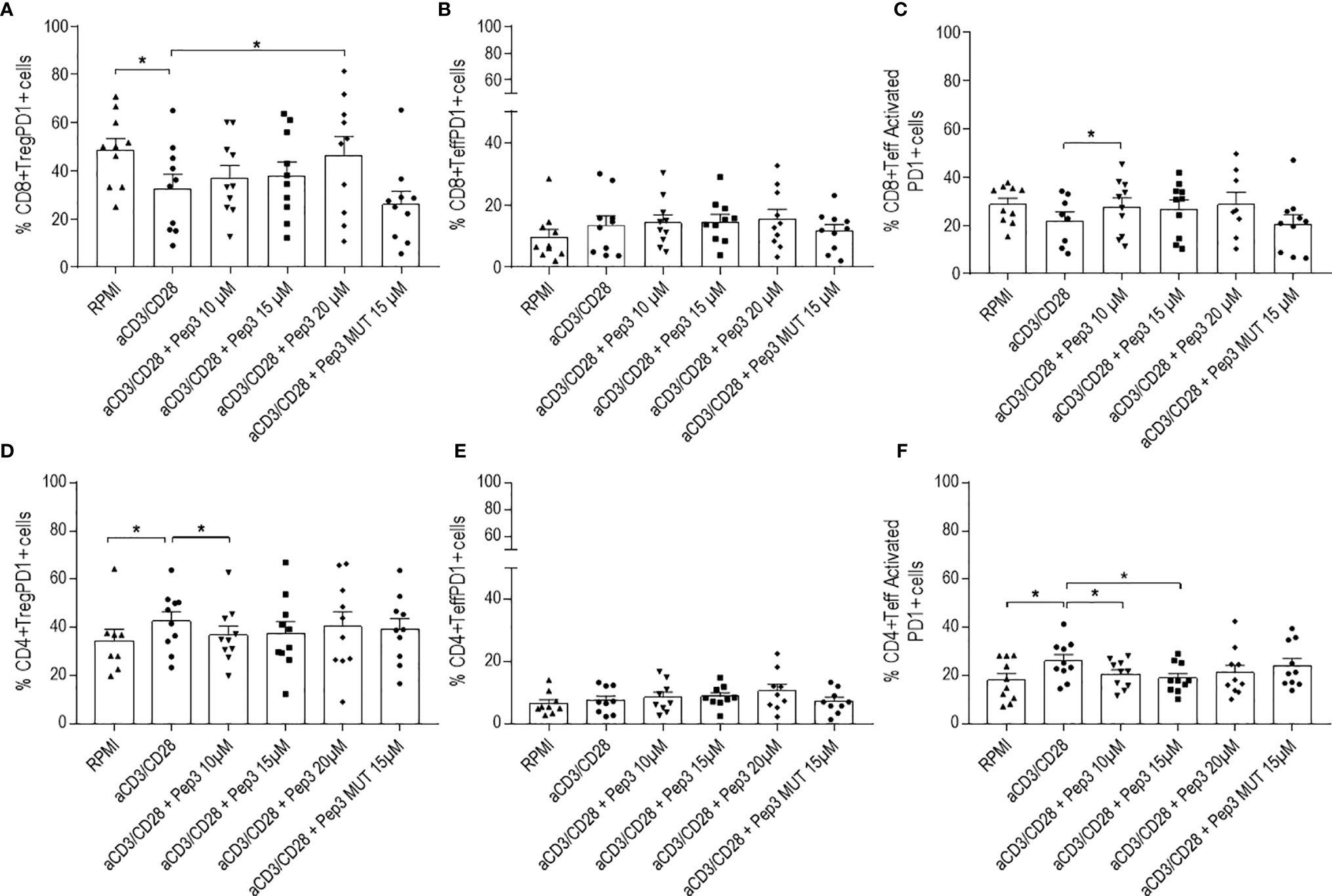
Figure 3 Frequency of CD8+PD1+ and CD4+PD1+ cell populations relative to thyroid cancer patients PBMC upon treatment with peptide 3 and subsequent stimulation with anti-CD3/CD28 beads for 4 days. Upper graphs show the percentages of CD8+ Treg PD1+ cells (A), CD8+ Teff PD1+ cells (B), CD8+ Teff activated PD1+ cells (C). Lower graphs show the percentages of CD4+ Treg PD1+ cells (D), CD4+ Teff PD1+ cells (E), CD4+ Teff activated PD1+ cells (F). Percentage of PD1+ cells was evaluated in comparison to the corresponding parental subset under evaluation. Statistical significance was evaluated using Wilcoxon matched-pairs signed rank test. Data are expressed as mean ± SEM, and each dot corresponds to an individual TC patient (n = 9-10). *p < 0.05.
Conversely, with regard to CD4+ T cells, Pep3 induced a decrease of CD4+ Teff activated PD1+ cells after 4 days of anti-CD3/CD28 stimulation (Figure 3F), while the total number of CD4+Teff PD1+ was not affected (Figure 3E). Pep3, at 10 µM, induced a reduction of the percentages of CD4+ Treg PD1+ (Figure 3D), although this effect did not persist until day 6 (Figure 4D).
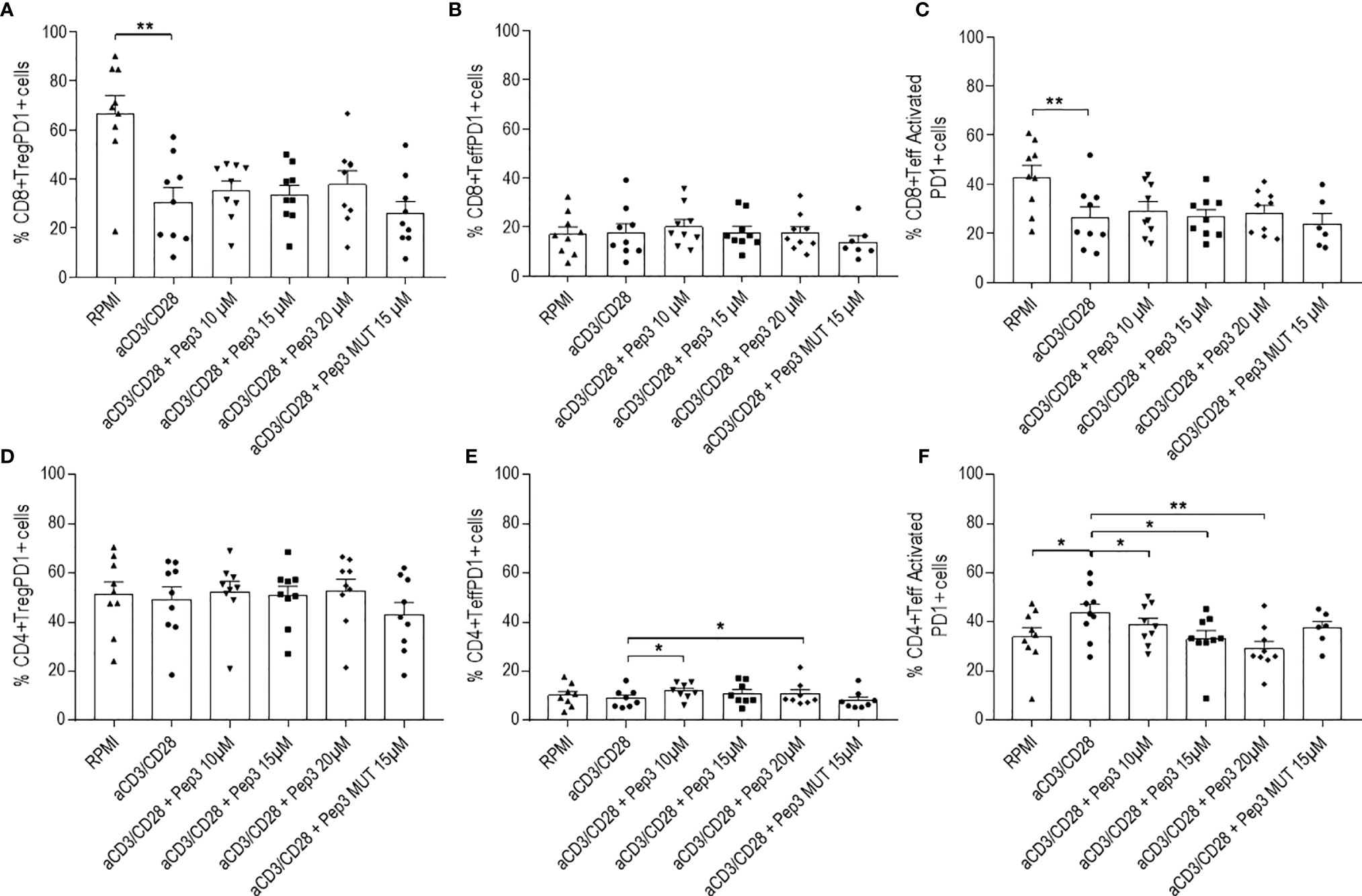
Figure 4 Frequency of CD8+PD1+ and CD4+PD1+ cell populations relative to thyroid cancer patients PBMC upon treatment with peptide 3 and subsequent stimulation with anti-CD3/CD28 beads for 6 days. Upper graphs show the percentages of CD8+ Treg PD1+ cells (A), CD8+ Teff PD1+ cells (B), CD8+ Teff activated PD1+ cells (C). Lower graphs show the percentages of CD4+ Treg PD1+ cells (D), CD4+ Teff PD1+ cells (E), CD4+ Teff activated PD1+ cells (F). Percentage of PD1+ cells was evaluated in comparison to the corresponding parental subset under evaluation. Statistical significance was evaluated using Wilcoxon matched-pairs signed rank test. Data are expressed as mean ± SEM, and each dot corresponds to an individual TC patient (n = 9). *p < 0.05 **p < 0.01.
After 6 days of stimulation, the effect of Pep3 on CD8+ Treg PD1+, CD8+ Teff PD1+, and CD8+ Teff activated PD1+ subsets was negligible (Figures 4A–C). Of note, a persistent reduction on the CD4+ Teff activated PD1+ in a more evident dose-dependent manner was detected (Figure 4F).
Consistently with the above results, comparing the effect of Pep3 pretreatment followed by 4 days of anti-CD3/CD28 stimulation in PBMC of TC cancer patients versus a small cohort of healthy donors (HD), no differences were observed in the analyzed cell subsets (Figure S4).
Discussion
In the area of immunotherapy, immunological checkpoints, such as CTLA-4, PD1, or PDL1, are membrane proteins involved in the immune response that, when inhibited, cause an increase in T-cell activity and a consequent antitumor effect. However, these checkpoint inhibitors can also cause adverse effects including autoimmune endocrinopathies, such as thyroid dysfunction and hypophysitis (1). Therefore, in cancer patients receiving anti-PD1, anti-PDL1, or anti-CTLA4 drugs, regular endocrine assessment is recommended to make early diagnosis and appropriate treatment.
This study aimed to elucidate whether p53 reactivation by peptide 3 inhibitor of the MDM2/MDM4 heterodimer (14) formation, whose administration is known to inhibit tumor growth, could have beneficial effects on tumor progression by restoring antitumoral immune responses whilst avoiding the development of autoimmune responses.
The effect of p53 reactivator on immunotypes in the peripheral blood of patients affected by thyroid carcinoma was investigated. It is interesting to remark that treatment, indeed, caused a decrease of T regulatory CD4+ and CD8+ percentages and an increase of T effector cells either after 4 and 6 days of anti-CD3/CD28 stimulation following Pep3 pretreatment. Indeed, these data are strongly at odds with the anticipated outcome of activating p53, which should be an increase of FOXP3+ Tregs as observed in other experimental models of autoimmunity (3, 19, 20) and in PBMC of rheumatoid arthritis (3) and T1D patients (21). The current results of this investigation envisage the potential effect of the new immunotherapy in stimulating the activity of CD4+ and CD8+ antitumor specific T lymphocytes either in the tumor-infiltrating microenvironment and at distant sites in the periphery. As regard to the potential side effect associated with the use of other immune checkpoint targeted drugs such as PD1, PDL1, and CTLA4 toward autoimmunity, it is important to underline that in parallel to the reduction of the Treg/Teff ratio, percentages of activated T effector cells were decreased in vitro. This envisages that this potential immunotherapy drug has a lower risk of autoimmunity development and may potentially control an already established thyroid autoimmune disease such as Hashimoto’s thyroiditis (HT). HT is frequently observed in thyroid cancer patients, and it may precede the tumor development since pediatric age (32, 33), as observed in the pediatric patient 12 of the ongoing investigation (Supplementary Table 1). Of note, the results of the present study could indeed open new perspectives for the treatment of children affected by TC eventually associated with HT as observed in patients recruited at our Hospital (Supplementary Table 1), and thus they are presently object of examination.
To date the prognostic significance of PD1/PDL1 levels of expression in tumor cells, immune cells, and tumor microenvironment is controversial (34, 35). The PD1 immune checkpoint halts later immune responses primarily in periphery by attenuating T-cell signaling downstream of the T-cell receptor (36). This avoids immune cell damage in pathogenic responses and suppresses autoimmunity. Consistently, Nishimura (37) first highlighted a correlation between PD1 pathway and the onset of autoimmunity. In fact, a defective activation of regulatory T cells was observed in Type 1 diabetes patients due to the lower expression of PD1 (25). In multiple cancer cell types, PDL1 expression seems to be associated with the patient’s prognosis and be a predictive tool for the response to PD1/PDL1 therapy. Hence, high levels of PD1 and PDL1 correlate with a favorable prognosis while low PD1/PDL1 expression with poorer cancer survival (34). In other studies, PD-L1 and PD-1 expression was significantly correlated with several adverse prognostic pathologic factors, including higher T-stage, diffuse Lauren histologic type, and lymphatic invasion (33). Furthermore, recent studies highlight the importance of quantitatively imaging PD1/PDL1 interactions in tumor samples from patients. Analysis across multiple patient cohorts revealed inter-cancer, inter-patient, and intra-tumoral heterogeneity on interacting immune checkpoints (38). Sánchez-Magraner et al. reported that within tumors selecting for an elevated level of PD1/PDL1 interaction, there is a greater dependence on this pathway for immune evasion and they show more impressive patient response to treatment (38).
In light of the foregoing, the PD1 expression on lymphocyte subsets under the effect of p53 activation was additionally evaluated. Although, PD1+CD8+Treg cells increased after 4 days of anti-CD3/CD28 stimulation, no effect was observed at the following time of 6 days on CD8+ Treg, even if a significant increase of activated CD8+ Teff PD1+ at 4 days was observed although negligible at 6 days. Of note, PD1+CD4+Treg had a tendency to decrease after 4 days in favor of an antitumor immune response. Nevertheless, this effect did not persist in the following time point, 6 days post treatment. Interestingly, as regard the CD4+ counterpart, the decrease of activated CD4+ Teff included a decrease of PD1+ CD4+ activated Teff subset as compared to CD8+ Teff PD1+ subset, suggesting a defective regulation in the initial activation stage, therefore potentially protective toward the autoimmunity progression.
As regard the putative mechanism of the effect of Pep3, upon CD3/CD28 stimulation, to decrease CD4+Treg PD1+ cells, one may hypothesize a role of sterol-regulatory-element-binding protein (SREBP) cleavage-activating-protein (SCAP) signaling that has been shown to link TCR engagement to PD1 expression (39). Moreover, SREBP/SCAP pathway controls the expression of enzymes for both fatty-acid synthesis and mevalonate metabolism. Thus, drugs such Simvastatin, which target the enzyme 3-hydroxy-3-methylglutaryl-CoA reductase (HMGCR) and the geranylgeranyl transferase type I inhibitor (GGT1)-2147 of PD1, could modulate PD1 expression on CD4+Treg. Further, p53 is known to repress the mevalonate pathway (40).
On a general ground, the molecular mechanisms underlying Pep3 activity on different target cells and in particular on T cells remain to be fully elucidated. The transcriptional program elicited by p53 differs depending on the cell type, activation status of the cells, and the microenvironment. In immune cells, FOXP3 represents a well-defined target of p53 (41). In a preliminary evaluation of the transcriptional program following Pep3 treatment on human healthy PBMC, a set of genes that are directly and indirectly related to p53 activity and involved in tumor and immune cell activation was investigated. Initial data showed increased mRNA levels of FOXP3 and IL 1β, a NF-kB target and a marker of antitumoral response (42), while no remarkable change of NF-kB1 mRNA levels, a Rel protein-specific transcription inhibitor, was observed. Although these results were achieved with a small number of samples and additional experiments are required to provide a clear and strong evidence, they may indicate a specific activity of p53 re-activation by Pep3 in HD PBMCs.
In conclusion, the present findings indicate the potential utility of p53 reactivation from a therapeutic perspective for treatment of autoimmunity and associated conditions of cancer and autoimmune diseases.
Data Availability Statement
The raw data supporting the conclusions of this article will be made available by the authors, without undue reservation.
Ethics Statement
The studies involving human participants were reviewed and approved by the Local Institutional Review Board (IRB) of the Bambino Gesù Children’s Hospital (OPBG) and Sapienza University. The patients/participants provided their written informed consent to participate in this study.
Author Contributions
AA, EG, and MRA conducted experiments. AS provided materials and clinical informations on patients. FM designed the molecular analysis and contributed to the design of the study and drafting the whole manuscript. EB and MMR critically revised the study and contributed to drafting of the manuscript. AG added clinical informations on pediatric patients. AF designed and supervised conduction of the study and wrote the main manuscript. All authors reviewed the manuscript. All authors contributed to the article and approved the submitted version.
Funding
This work was supported by the Italian Ministry of Health Ricerca Corrente RC2020_INFETT_FIERABRACCI (AF) and by the Italian Association for Cancer Research Grant AIRC IG 21814 (FM).
Conflict of Interest
The authors declare that the research was conducted in the absence of any commercial or financial relationships that could be construed as a potential conflict of interest.
Publisher’s Note
All claims expressed in this article are solely those of the authors and do not necessarily represent those of their affiliated organizations, or those of the publisher, the editors and the reviewers. Any product that may be evaluated in this article, or claim that may be made by its manufacturer, is not guaranteed or endorsed by the publisher.
Acknowledgments
We thank Ms. Anna Lorusso (Children’s Hospital Bambino Gesù, Rome, Italy) for technical assistance in cell culture.
Supplementary Material
The Supplementary Material for this article can be found online at: https://www.frontiersin.org/articles/10.3389/fimmu.2021.728381/full#supplementary-material
References
1. Gianchecchi E, Fierabracci A. Inhibitory Receptors and Pathways of Lymphocytes: The Role of PD-1 in Treg Development and Their Involvement in Autoimmunity Onset and Cancer Progression. Front Immunol (2018) 9:2374. doi: 10.3389/fimmu.2018.02374
2. Zitvogel L, Tesniere A, Kroemer G. Cancer Despite Immunosurveillance: Immunoselection and Immunosubversion. Nat Rev Immunol (2006) 6:715–27. doi: 10.1038/nri1936
3. Park JS, Lim MA, Cho ML, Ryu JG, Moon YM, Jhun JY, et al. P53 Controls Autoimmune Arthritis via STAT-Mediated Regulation of the Th17 Cell/Treg Cell Balance in Mice. Arthritis Rheumatol (2013) 65:949–59. doi: 10.1002/art.37841
4. Kim HR, Park HJ, Ha SJ. PD-1: Dual Guard for Immunopathology. Oncotarget (2015) 6:21783–4. doi: 10.18632/oncotarget.4787
5. Iwai Y, Hamanishi J, Chamoto K, Honjo T. Cancer Immunotherapies Targeting the PD-1 Signaling Pathway. J Biomed Sci (2017) 24(1):26. doi: 10.1186/s12929-017-0329-9
6. Ahmadzadeh M, Johnson LA, Heemskerk B, Wunderlich JR, Dudley ME, White DE, et al. Tumor Antigen-Specific CD8 T Cells Infiltrating the Tumor Express High Levels of PD-1 and Are Functionally Impaired. Blood (2009) 114:1537–44. doi: 10.1182/blood-2008-12-195792
7. Kumagai S, Togashi Y, Kamada T, Sugiyama E, Nishinakamura H, Takeuchi Y, et al. The PD-1 Expression Balance Between Effector and Regulatory T Cells Predicts the Clinical Efficacy of PD-1 Blockade Therapies. Nat Immunol (2020) 21:1346–58. doi: 10.1038/s41590-020-0769-3
8. Park HJ, Kusnadi A, Lee EJ, Kim WW, Cho BC, Lee IJ, et al. Tumor-Infiltrating Regulatory T Cells Delineated by Upregulation of PD-1 and Inhibitory Receptors. Cell Immunol (2012) 278:76–83. doi: 10.1016/j.cellimm.2012.07.001
9. Riley RS, June CH, Langer R, Mitchell MJ. Delivery Technologies for Cancer Immunotherapy. Nat Rev Drug Discov (2019) 18:175–96. doi: 10.1038/s41573-018-0006-z
10. Xu Y, Fu Y, Wang J, Zhang B. Predictive Biomarkers of Immune Checkpoint Inhibitors-Related Toxicities. Front Immunol (2020) 11:2023. doi: 10.3389/fimmu.2020.02023
11. Wolchok JD, Kluger H, Callahan MK, Postow MA, Rizvi NA, Lesokhin AM, et al. Nivolumab Plus Ipilimumab in Advanced Melanoma. N Engl J Med (2013) 369(2):122–33. doi: 10.1056/NEJMoa1302369
12. Postow MA, Chesney J, Pavlick AC, Robert C, Grossmann K, McDermott D, et al. Nivolumab and Ipilimumab Versus Ipilimumab in Untreated Melanoma. N Engl J Med (2015) 372(21):2006–17. doi: 10.1056/NEJMoa1414428
13. Fierabracci A, Pellegrino M. The Double Role of P53 in Cancer and Autoimmunity and Its Potential as Therapeutic Target. Int J Mol Sci (2016) 17(12):1975. doi: 10.3390/ijms17121975
14. Pellegrino M, Mancini F, Lucà R, Coletti A, Giacchè N, Manni I, et al. Targeting the MDM2/MDM4 Interaction Interface as a Promising Approach for P53 Reactivation Therapy. Cancer Res (2015) 75(21):4560–72. doi: 10.1158/0008-5472
15. Linke K, Mace PD, Smith CA, Vaux DL, Silke J, Day CL. Structure of the MDM2/MDMX RING Domain Heterodimer Reveals Dimerization Is Required for Their Ubiquitylation in Trans. Cell Death Differ (2008) 15(5):841–8. doi: 10.1038/sj.cdd.4402309
16. Okamoto K, Taya Y, Nakagama H. Mdmx Enhances P53 Ubiquitination by Altering the Substrate Preference of the Mdm2 Ubiquitin Ligase. FEBS Lett (2009) 583(17):2710–4. doi: 10.1016/j.febslet.2009.07.021
17. Levrero M, De Laurenzi V, Costanzo A, Gong J, Wang JY, Melino G. The P53/P63/P73 Family of Transcription Factors: Overlapping and Distinct Functions. J Cell Sci (2000) 113(Pt 10):1661–70. doi: 10.1242/jcs.113.10.1661
18. Green DR, Kroemer G. Cytoplasmic Functions of the Tumour Suppressor P53. Nature (2009) 458(7242):1127–30. doi: 10.1038/nature07986
19. Muñoz-Fontela C, Mandinova A, Aaronson SA, Lee SW. Emerging Roles of P53 and Other Tumour-Suppressor Genes in Immune Regulation. Nat Rev Immunol (2016) 16(12):741–50. doi: 10.1038/nri.2016.99
20. Takatori H, Kawashima H, Suzuki K, Nakajima H. Role of P53 in Systemic Autoimmune Diseases. Crit Rev Immunol (2014) 34(6):509–16. doi: 10.1615/critrevimmunol.2014012193
21. Pellegrino M, Traversi G, Arena A, Cappa M, Rosado MM, Andreani M, et al. Effect of P53 Activation Through Targeting MDM2/MDM4 Heterodimer on T Regulatory and Effector Cells in the Peripheral Blood of Type 1 Diabetes Patients. PloS One (2020) 15(1):e0228296. doi: 10.1371/journal.pone.0228296
22. Nagayama Y. Thyroid Autoimmunity and Thyroid Cancer - The Pathogenic Connection: A 2018 Update. Horm Metab Res (2018) 50(12):922–31. doi: 10.1055/a-0648-4593
23. Laha D, Nilubol N, Boufraqech M. New Therapies for Advanced Thyroid Cancer. Front Endocrinol (Lausanne) (2020) 11:82. doi: 10.3389/fendo.2020.00082
24. Manca Bitti ML, Saccucci P, Capasso F, Piccinini S, Angelini F, Rapini N, et al. Genotypes of P53 Codon 72 Correlate With Age at Onset of Type 1 Diabetes in a Sex-Specific Manner. J Pediatr Endocrinol Metab (2011) 24(7-8):437–9. doi: 10.1515/jpem.2011.058
25. Perri V, Russo B, Crinò A, Schiaffini R, Giarda E, Cappa E, et al. Expression of PD-1 Molecule on Regulatory T Lymphocytes in Patients With Insulin-Dependent Diabetes Mellitus. Int J Mol Sci (2015) 16(9):22584–605. doi: 10.3390/ijms160922584
26. Gianchecchi E, Crinò A, Giorda E, Perri V, Luciano R, Lo Russo A, et al. Altered B Cell Homeostasis and Toll-Like Receptor 9-Driven Response in Type 1 Diabetes Carriers of the C1858T PTPN22 Allelic Variant: Implications in the Disease Pathogenesis. PloS One (2014) 9(10):e110755. doi: 10.1371/journal.pone.0110755
27. Pellegrino M, Crinò A, Rosado MM, Fierabracci A. Identification and Functional Characterization of CD8+ T Regulatory Cells in Type 1 Diabetes Patients. PloS One (2019) 14(1):e0210839. doi: 10.1371/journal.pone.0210839
28. Churlaud G, Pitoiset F, Jebbawi F, Lorenzon R, Bellier B, Rosenzwajg M, et al. Human and Mouse CD8(+)CD25(+)FOXP3(+) Regulatory T Cells at Steady State and During Interleukin-2 Therapy. Front Immunol (2015) 6:171. doi: 10.3389/fimmu.2015.00171
29. Huang CT, Workman CJ, Flies D, Pan X, Marson AL, Zhou G, et al. Role of LAG-3 in Regulatory T Cells. Immunity (2004) 21(4):503–13. doi: 10.1016/j.immuni.2004.08.010
30. Prodosmo A, Giglio S, Moretti S, Mancini F, Barbi F, Avenia N, et al. Analysis of Human MDM4 Variants in Papillary Thyroid Carcinomas Reveals New Potential Markers of Cancer Properties. J Mol Med (Berl) (2008) 86(5):585–96. doi: 10.1007/s00109-008-0322-6
31. Dumont P, Leu JI, Della Pietra AC 3rd, George DL, Murphy M. The Codon 72 Polymorphic Variants of P53 Have Markedly Different Apoptotic Potential. Nat Genet (2003) 33(3):357–65. doi: 10.1038/ng1093
32. Liu Y, Li C, Zhao W, Wang Y. Hashimotos Thyroiditis Is an Important Risk Factor of Papillary Thyroid Microcarcinoma in Younger Adults. Horm Metab Res (2017) 49(10):732–8. doi: 10.1055/s-0043-117892
33. Chen YK, Lin CL, Cheng FT, Sung FC, Kao CH. Cancer Risk in Patients With Hashimoto’s Thyroiditis: A Nationwide Cohort Study. Br J Cancer (2013) 109:2496–501. doi: 10.1038/bjc.2013.597
34. Chang H, Jung WY, Kang Y, Lee H, Kim A, HK K, et al. Programmed Death-Ligand 1 Expression in Gastric Adenocarcinoma Is a Poor Prognostic Factor in a High CD8+ Tumor Infiltrating Lymphocytes Group. Oncotarget (2016) 7(49):80426–34. doi: 10.18632/oncotarget.12603
35. Hu FF, Liu CJ, Liu L, Zhang Q, Guo AY. Expression Profile of Immune Checkpoint Genes and Their Roles in Predicting Immunotherapy Response. Brief Bioinform (2020) 19:bbaa176. doi: 10.1093/bib/bbaa176
36. Okazaki T, Honjo T. The PD-1-PD-L Pathway in Immunological Tolerance. Trends Immunol (2006) 27(4):195–201. doi: 10.1016/j.it.2006.02.001
37. Nishimura H, Nose M, Hiai H, Minato N, Honjo T. Development of Lupus-Like Autoimmune Diseases by Disruption of the PD-1 Gene Encoding an ITIM Motif-Carrying Immunoreceptor. Immunity (1999) 11(2):141–51. doi: 10.1016/s1074-7613(00)80089-8
38. Sánchez-Magraner L, Miles J, Baker CL, Applebee CJ, Lee DJ, Elsheikh S, et al. High PD-1/PD-L1 Checkpoint Interaction Infers Tumor Selection and Therapeutic Sensitivity to Anti-PD-1/PD-L1 Treatment. Cancer Res (2020) 80(19):4244–57. doi: 10.1158/0008-5472.CAN-20-1117
39. Lim SA, Wei J, Nguyen TM, Shi H, Su W, Palacios G, et al. Lipid Signalling Enforces Functional Specialization of Treg Cells in Tumours. Nature (2021) 591(7849):306–11. doi: 10.1038/s41586-021-03235-6
40. Moon SH, Huang CH, Houlihan SL, Regunath K, Freed-Pastor WA, Morris JP4, et al. P53 Represses the Mevalonate Pathway to Mediate Tumor Suppression. Cell (2019) 176(3):564–580.e19. doi: 10.1016/j.cell.2018.11.011
41. Zhang D, Chen Y, Chen L, Yang R, Wang L, Liu W, et al. Ultraviolet Irradiation Promotes FOXP3 Transcription via P53 in Psoriasis. Exp Dermatol (2016) 25(7):513–8. doi: 10.1111/exd.12942
Keywords: p53, T regulatory cells, T effector cells, thyroid cancer, thyroiditis, immunotherapy, pediatric disease
Citation: Arena A, Stigliano A, Belcastro E, Giorda E, Rosado MM, Grossi A, Assenza MR, Moretti F and Fierabracci A (2021) p53 Activation Effect in the Balance of T Regulatory and Effector Cell Subsets in Patients With Thyroid Cancer and Autoimmunity. Front. Immunol. 12:728381. doi: 10.3389/fimmu.2021.728381
Received: 21 June 2021; Accepted: 09 August 2021;
Published: 30 August 2021.
Edited by:
Ciriaco A. Piccirillo, McGill University, CanadaReviewed by:
Jeffrey Louis Curtis, University of Michigan, United StatesRobert Weil, INSERM U1135 Centre d’Immunologie et de Maladies Infectieuses, France
Copyright © 2021 Arena, Stigliano, Belcastro, Giorda, Rosado, Grossi, Assenza, Moretti and Fierabracci. This is an open-access article distributed under the terms of the Creative Commons Attribution License (CC BY). The use, distribution or reproduction in other forums is permitted, provided the original author(s) and the copyright owner(s) are credited and that the original publication in this journal is cited, in accordance with accepted academic practice. No use, distribution or reproduction is permitted which does not comply with these terms.
*Correspondence: Alessandra Fierabracci, alessandra.fierabracci@opbg.net