Arbuscular mycorrhizal fungi inoculation exerts weak effects on species- and community-level growth traits for invading or native plants under nitrogen deposition
- 1College of Landscape Architecture and Forestry, Qingdao Agricultural University, Qingdao, China
- 2Shandong Peanut Research Institute/Chinese National Peanut Engineering Research Center, Shandong Academy of Agricultural Sciences, Qingdao, China
Nitrogen deposition and biological invasion are two major components of global environmental change. Nitrogen deposition has been considered to enhance the resource availability of recipient habitats, which influences the invasiveness of plant invader and the invasibility of recipient native communities. Nitrogen deposition has been shown to reduce the relative abundances of arbuscular mycorrhizal fungi (AMF) globally. AMF have been found to mutualistically symbiose with approximately 75% of plant species and act as a nutrient supplier. AMF may modify the structure of native plant communities, collaborate with alien plant invaders and thus promote their invasion. The alien woody invader, Rhus typhina L. has been introduced into North China as a horticultural species, invaded the native plant community and outperformed the native competitors in growth and in photosynthetic efficiency. Nevertheless, little is known about if nitrogen deposition and AMF inoculation synergistically alter the invasibility of native plant community. In this study, R. typhina was subjected to the artificial plant community assembled by four co-existing native species – Chenopodium album L., Vitex negundo var. heterophylla (Franch.) Rehd., Rhus chinensis Mill. and Acer truncatum Bunge in a mesocosm experiment. Nitrogen deposition and AMF inoculation were simulated as environmental and biotic filters respectively. Aboveground biomass and biomass proportion, reflecting plant growth and performance, and specific leaf area and chlorophyll concentration correlated with carbon use and photosynthetic capacity of both the alien invader and the native plants were measured and calculated after harvest. We found that AMF inoculation did not alter the trait variation of alien and native species to increasing nitrogen deposition level in general, although AMF inoculation impeded the increase of aboveground biomass for C. album, V. negundo and native community with increasing nitrogen deposition level. In the scenario of nitrogen deposition and AMF inoculation, a stable status of invasion dynamic may be maintained and needs to be checked with integration of traits at extended temporal scale.
Introduction
As the two crucial components of global environmental change, nitrogen (N) deposition and biological invasion have been boosted by anthropogenic activities and have inestimable effects on recipient ecosystems (Vitousek, 1992; Millennium Ecosystem Assessment, 2005; Borer and Stevens, 2022; Lopez et al., 2022). The global N deposition rate has been predicted to increase 1–2 folds by the middle of the 21st century (Jansson and Hofmockel, 2020). Multiple ecosystems have transformed from N deficiency to N saturation (Penuelas et al., 2020). China has become the third largest N deposition area and annual average N deposition rate has reached 20.4 kg.hm−2 (Valliere et al., 2017; Yu et al., 2019). N deposition has caused an alteration of the global nitrogen cycle and acts as an environmental stress in modifying the structure and function of terrestrial ecosystems, for instance species loss, degradation of biodiversity and homogenization of local flora (Hayes et al., 2019; Peng et al., 2019).
Likewise, biological invasion has been shown to have detrimental ecological effects such as the exclusion of native species and the alteration of community structure (Zhang and van Kleunen, 2019). Importantly, plant invasion success can be mediated through background nitrogen deposition across a range of different ecosystems (Valliere et al., 2017; Wang et al., 2021; Liu et al., 2022; Wang et al., 2022). Indeed, the resource fluctuation hypothesis predicts that N deposition may enhance the habitat resource availability and improve their hospitability for plant invasion (Davis et al., 2000). In view of this prediction, many studies have shown that N deposition promotes plant performance through a range of metrics, including biomass accumulation and photosynthetic efficiency for invading species (Wang et al., 2017; Wang et al., 2022; Xing et al., 2022). In particular, specific leaf area (SLA) and chlorophyll concentration have been commonly used to indicate photosynthetic efficiency (Wang et al., 2023). Since the leaf trait economic spectrum predicts a positive correlation between SLA and leaf N concentration and N is a constituent of chlorophyll, N deposition likely enhance SLA and chlorophyll concentration (Wang et al., 2017; Wang et al., 2022). However, the effects of N deposition on the invasibility of native plant communities have been mostly tested using herbs or field trials (Maron and Marler, 2008; Liu et al., 2018; Cavieres, 2021). Successful invasions are able to overcome the biotic barriers associated with the recipient community, and the underlying mechanisms such as competitive exclusion and allelopathy are contributors (Young et al., 2022). N deposition also influences the performance of the native plant community, the construction of biotic resistance and thus the invasibility of the native plant community (Li et al., 2022a). Several studies have proposed that N deposition may have stressful effects on the diversity and/or productivity of native plant communities and reduce the biotic resistance of the native plant community to invasion (Zheng et al., 2020; Gao et al., 2021). Other studies have suggested the opposite, as N deposition promoted the growth of native plants, enhanced the productivity of native plant communities and benefited biotic resistance (Wang et al., 2021; Liu et al., 2022). Therefore, the plant–plant interactions including alien vs. native and native vs. native both affect the invasion dynamic.
Arbuscular mycorrhizal fungi (AMF) can symbiose with approximately 75% of vascular plants: with aid in nutrient assimilation, water uptake, pathogen defense and stress tolerance (Smith and Read, 2008; Wipf et al., 2019; Frew et al., 2020). In exchange, AMF obtain carbon sources such as carbohydrates and lipids from plants (Jiang et al., 2017). Despite the above benefits, the net effects of AMF on plant performance are uncertain when considering invasive and native plants integratively. Several studies have shown that AMF have a higher colonization rate on invasive plants and promote their performance (Dong et al., 2021; Lamit et al., 2022; Sun et al., 2022). Thus, a competitive advantage of invasive plants over native plants can be realized (Lamit et al., 2022; Sun et al., 2022). However, a meta-analysis has reported that AMF colonization did not differ between invasive and native plants and invasive plants possibly do not symbiose with AMF or are poorly colonized and benefit less from AMF than native plants (Bunn et al., 2015). Cheng et al. (2019) have also shown that the inoculation of arbuscular mycorrhizal fungi has the potential to help the native species Bidens biternata (Lour.) Merr. et Sherff resist the invasion of Bidens alba. (L.) DC. Therefore, the plant–plant interaction between alien invader and recipient native plant community is ambiguous when facing AMF colonization and the effects of AMF inoculation on the invasibility of native plant community are uncertain.
The introduction of alien woody plant species appears to be inevitable considering the improvement of aesthetic value for urban landscapes and the economic benefits derived from forestry production (Richardson and Rejmánek, 2011). However, the intentional introduction of alien woody species has led to their invasion and has caused severe ecological safety problems to both the artificial ecosystem and natural ecosystem such as the threat to native biodiversity and biotic homogenization (Richardson et al., 2014). Rhus typhina L. has been commonly used as an ornamental tree species for urban greening and has been cultivated into the forest plantation in North China (Wang et al., 2008). Since this species has strong allelopathic effects and the dispersal of this species may alter the native plant community structure, the invasive potential of this species has been considered to be great (Xu et al., 2023). Prior mesocosm studies have shown that R. typhina outperforms its native counterparts (e.g. Quercus acutissima Carr., Sapindus mukorossi Gaertn., Cotinus coggygria Scop. and Rhus chinensis Mill.) in growth (e.g. biomass accumulation) and traits associated with resource use (e.g. specific leaf area and chlorophyll concentration) (Yuan et al., 2013; Wang et al., 2016; Guo et al., 2020; Wang et al., 2022). Especially, N deposition confers a competitive advantage on R. typhina (Yuan et al., 2013; Wang et al., 2022). However, few previous studies on R. typhina considered the invasibility of the recipient native plant community, despite several prior studies on herbaceous plant invasion examining the probable biotic resistance of artificial native herb communities (Liu et al., 2018; Jin et al., 2022; Li et al., 2022b). The present study subjected the alien woody invader, Rhus typhina L. to the artificial plant community assembled by four co-occurring native species – Chenopodium album L., Vitex negundo var. heterophylla (Franch.) Rehd., Rhus chinensis Mill. and Acer truncatum Bunge in a mesocosm experiment. By evaluating the probable phenotypic variation of the alien invader and native plant community in response to different treatments of nitrogen deposition and AMF inoculation, the present study aimed to explore the probable effects of nitrogen deposition and AMF inoculation on shaping the invasibility of the native plant community.
Materials and methods
Study site and plant materials
The experiment was conducted in a greenhouse of Qingdao Agricultural University, Qingdao city, Shandong Province, China (36° 31′ N, 120° 39′ E), the area has a warm temperate monsoon climate, with an average temperature of 12.7 °C and an average annual precipitation of 700 ± 100 mm (Wang et al., 2022). The greenhouse was covered with plastic film.
The seeds of R. typhina, V. negundo, R. chinensis and A. truncatum were sampled from Mount Lao (36° 19′ N, 120° 62′ E) in November, 2020 and the seeds were stored at 0–4 °C during the winter. The following actions were taken to stimulate germination in April–May, 2021. The seeds of R. chinensis and R. typhina were washed with 70 °C baking soda solution for 10 minutes and then washed with tap water. The seeds of R. typhina, V. negundo, R. chinensis and A. truncatum were soaked in tap water for 24 hours respectively. The soaked seeds of each species were then placed on trays to promote germination for 7–14 days. During this period, we sprayed water into the tray every morning and noon to keep all the seeds moist and cover the seeds with a layer of gauze to reduce water loss (Wang et al., 2022). After germination, healthy and strong seedlings of each species with 4–6 leaves were selected and transplanted into plug plates. When most seedlings of each species in the plug plates had four fully-developed leaves, we collected C. album seedlings with four fully-developed leaves at the campus of Qingdao Agricultural University. Seedlings of five species with similar size and four leaves were transplanted into plastic pots (200 mm in diameter and 200 mm in height) for further treatments.
Each pot was loaded with a mixture of 1:1 (v/v) of sandy air-dried loam (collected locally) and peat (specification: 10–30 mm, pindstrup substrate, Denmark). The total weight of the substrate was 5.0 kg. Debris and stones were removed from the sandy loam beforehand. Autoclaving was performed to kill all soil biota before treatments.
Experimental design
We grew the invasive R. typhina as target species in pot-mesocosms with a synthetic native resident community. One R. typhina seedling was planted in the middle of the pot. The other four native plant species (C. album, V. negundo, R. chinensis and A. truncatum), one seedling per species, were planted around the R. typhina seedling (see Supplementary Material 1). This planting pattern was designed to explore the effects of native plant interaction on the performance of exotic R. typhina. Rhus chinensis and A. truncatum are trees, while V. negundo and C. album are shrub and forb, respectively. All the four species, which co-exist with R. typhina in the field, are representative native species in the study region. Frequent inter-species interaction such as competition between R. typhina and the four native counterparts is expected.
A two-factor experiment was conducted with three N deposition levels and two arbuscular mycorrhizal fungi (AMF) inoculation levels. The three N deposition levels were 0, 6 and 12 g N m−2y−1 (designated as N0, N6 and N12, respectively), representing no deposition, deposition level already recorded in some areas of northern and southern China (Lü and Tian, 2007; Zhang et al., 2011) and deposition level that may be reached in the future (Luo et al., 2014), respectively. N loading was simulated by adding an aqueous ammonium nitrate (NH4NO3) solution directly to the soil every 2 weeks. The solutions were prepared with deionized water. The amount of NH4NO3 used was calculated based on the above-mentioned N addition levels. Within each N deposition level, two AMF inoculation treatments were employed: R. typhina and the native resident community inoculated with AMF (+ AMF) and non-inoculated controls (0 AMF). Funneliformis mosseae, Glomus versiforme and Rhizophagus intraradices were selected and combined (1:1:1) as AMF inoculation treatment since they are commonly distributed in Qingdao area in our field survey, and all the selected plant species can be successfully colonized by the combination of the three AMF species according to our pre-experiment. The AMF for inoculation treatment were provided by the Institute of Mycorrhizal Biotechnology, Qingdao Agricultural University. Each plant received 8 g inocula (approximately 320 AMF spores) for the AMF inoculation treatment. The same amount of autoclaved inocula were added to every plant as non-inoculated controls. As such, the experiment included 6 treatments, and 6 replicates were included at each treatment, resulting in a total of 36 pots.
Insects and weeds were controlled manually. Insect pests were also controlled with sticky boards. All pots received adequate watering. The temperature and humidity were measured using DL-TH20 digital display thermometer and hygrometer (Hangzhou Gsome Technology Co., Hangzhou, China). The average temperature and relative humidity in the greenhouse during the experiment were 26.9 ± 4.8 °C and 75% ± 19% respectively (n = 4091, from 18:39, July 2nd to 23:39, September 25th, recorded every 30 min; typical diurnal pattern of temperature and humidity see Supplementary Material 2). The light intensity was measured using Quantum1/Foot Candle Meter (Spectrum Technologies, Inc., Aurora, IL, USA). The light intensity inside the greenhouse was 78.3% ± 0.033 (n = 6) of the outdoor light intensity. The position of the pots was re-randomized weekly. The study was carried out from July 1st to September 25th, 2021, and it lasted for 87 days.
Harvest and measurements
All plants survived during the experimental period and were measured and harvested on September 25th, 2021. Plant height and crown area were measured for each plant individual in each treatment. Plant height was gauged as the vertical distance between base and apex. The crown area was calculated as (Wang et al., 2022):
a denotes the maximal horizontal length of the plant crown, while b denotes the width of the plant crown perpendicular to a.
The average leaf area was measured with 10 fully expanded leaves from each treatment (third or/and fourth leaves from the tip). A scanner (Epson Perfection V700, Seiko Epson, Japan) was used to scan the leaves, and then Image J (National Institutes of Health, Maryland, USA) was used to calculate the leaf area. Subsequently, the leaves were oven-dried for 0.5 h at 105 °C to inactivate enzymes, then at 80 °C for 48 h, and leaf dry weight (LDW) was weighed using an electronic balance (Ohaus AR1140, Ohaus Corporation, USA). The specific leaf area (SLA) was calculated as: leaf area/leaf dry weight.
One leaf on the third leaf node from the tip of each plant individual was selected for the determination of chlorophyll concentration (CHL) using the ethanol method. Approximately 0.1 g of fresh leaf tissue was ground in 10 ml of 95% ethanol with calcium carbonate and quartz sand until all the leaf tissue dissolved. The absorbances of the supernatant were measured at 649 nm (A649) and 665 nm (A665) using a spectrophotometer after 24 h of dark treatment. Chlorophyll a concentration (Chla), chlorophyll b concentration (Chlb) and CHL were calculated separately as (Wang et al., 2019):
The aboveground part of seedlings of each species was then harvested and divided into leaf and stem. The root of each species was not harvested partly because their roots intertwined with each other and is difficult to distinguish, and partly because the above-ground competition rather than below-ground competition mainly impacts the species dominance (Kiaer et al., 2013). The leaves and stem were separated and dried at 105 °C for 0.5 h to inactivate enzymes, and then dried at 80 °C for 48 h. After that, the biomass of leaves and stem for each species and each replicate were weighed using an electronic balance (Ohaus AR1140, Ohaus Corporation, USA). The aboveground biomass of each species and each replicate was calculated as the sum of leaf mass and stem mass. The aboveground biomass of the native plant community of each replicate was calculated as the sum of the aboveground biomass for each native plant including C. album, V. negundo, R. chinensis and A. truncatum. The biomass proportion of R. typhina was calculated as: aboveground biomass of R. typhina/(aboveground biomass of R. typhina + aboveground biomass of native plant community) × 100%, while the biomass proportion of the native plant community was calculated as: aboveground biomass of native plant community/(aboveground biomass of R. typhina + aboveground biomass of native plant community) × 100%.
The community-weighted mean (CWM) of SLA and CHL each for the native plant community was calculated as the average trait value in the community weighted by the important value of each species, which evolves from the method of Garnier et al. (2004). The calculation process of the CWM for a single trait is as follows:
The above equation is developed from the method of Curtis and McIntosh (1951). Ar represents the relative abundance of a single species, which is the ratio of the abundance of a single species to the total abundance of the species in the community, in the present study the Ar is 1/4 for each native species. Cr represents the relative coverage of a single species, which is the ratio of the coverage of a single species to the total coverage of the species in the community. Since coverage reflects the vertically projected area of the plant canopy to a large extent, the crown area is used to represent coverage in the present study. Hr represents the relative height of a single species, which is the ratio of the height of a single species to the total height of the species in the community.
Where the CWMtrait represents the community-weighted mean of a single trait (SLA or CHL for the present study), the s is the species number of the community (s = 4 for the present study), the IVi is the important value of the ith species in the community and the ti is the trait value of the ith species in the community.
The infection rate of AMF was measured following the method by Zhao et al. (2023). The roots of each species each replicate were fragmented into 0.5–1 cm-long slices and then the sliced were placed into a test tube with a KOH solution with a 5%–10% concentration Then, the test tube was water bathed for 20–60 min at 90 °C. After water bath, the roots were soaked in the 2% concentration of HCL solution for 5 min. The roots were stained using with a 0.01% concentration of acid fuchsin stain after the acid was removed from the roots. Then, the stained roots were water bathed for 20–60 min at 90 °C. Color separation was done on the roots with lactic acid. Then, microscopic observation was used. The AMF infection rate was calculated as follows:
n0 represents the root slice number without AMF colonization, n1 represents the root slice number with an AMF colonization rate of 10%, n2 represents the root slice number with an AMF colonization rate of 20%, n10 represents the root slice number with an AMF colonization rate of 100%.
Data analysis
SLA was log(x)-transformed and the biomass proportions of R. typhina and native plants were log(x+1)-transformed (Isles, 2020). Thus, all trait data met the assumption of normality and homogeneity of variance prior to analysis. A one-way analysis of variance (ANOVA) was performed to test the effects of nitrogen deposition on the infection rate of AMF for different species. A two-way analysis of variance (ANOVA) was applied to test the effects of nitrogen deposition and AMF inoculation on aboveground biomass, biomass proportion, SLA and CHL of R. typhina the native plant community. If a significant treatment effect was detected, post hoc pair-wise comparisons of means were performed to examine the differences between treatments using Tukey’s test for multiple comparisons. The correlations between nitrogen deposition level and plant performance including aboveground biomass, biomass proportion, SLA and CHL for each species and native plant community were detected using linear regression under different conditions of AMF inoculation. All data analyses were conducted using SPSS 25.0 (IBM Corp., Armonk, NY, USA).
Results
All plant species were infected by the AMF (Figure 1). The infection rate of R. typhina decreased with increasing nitrogen deposition (Figure 1A). Chenopodium album showed the highest infection rate without nitrogen deposition and nitrogen deposition decreased its infection rate (Figure 1B). Both V. negundo and R. chinensis displayed an increase in infection rate with increasing nitrogen deposition (Figures 1C, D). The infection rate of A. truncatum was lowest in the treatment of N6, while a significantly higher infection rate was shown in the treatment of N12 (Figure 1E).
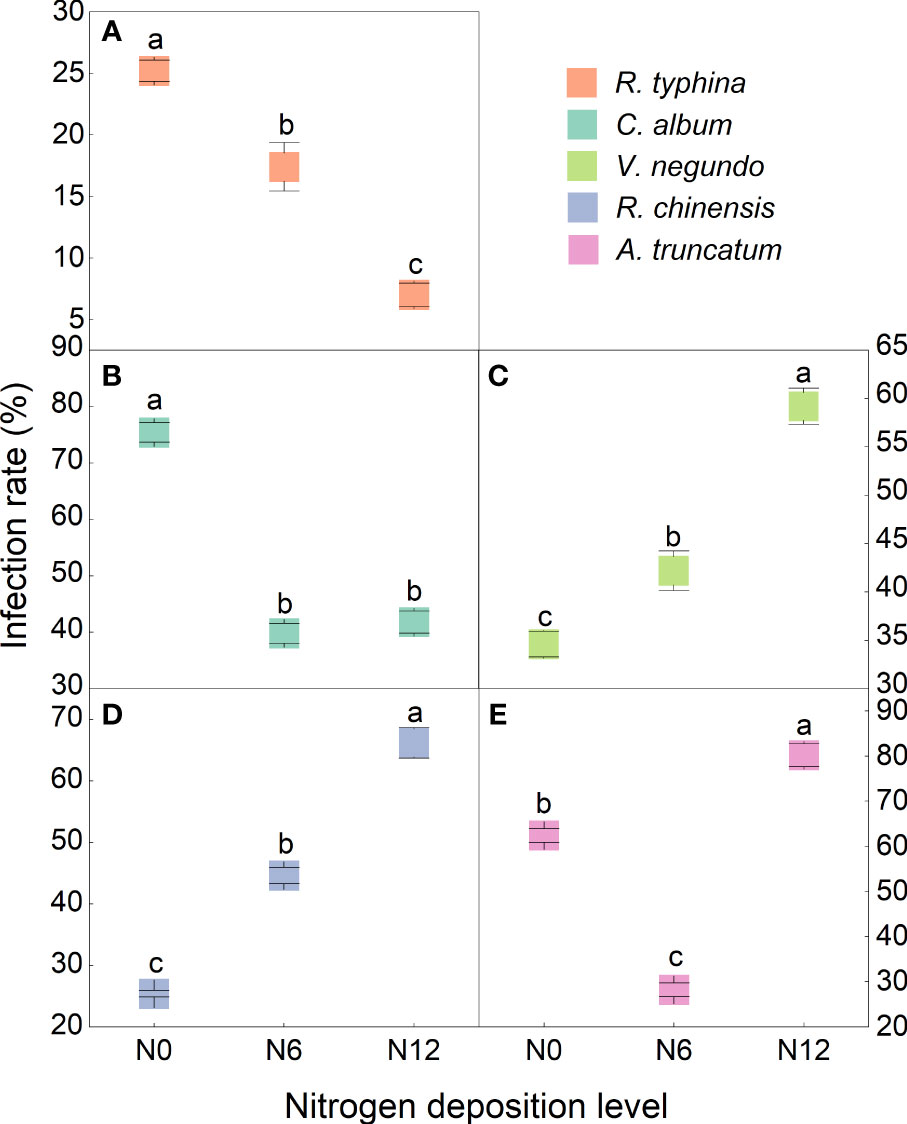
Figure 1 Variation in the arbuscular mycorrhizal fungi (AMF) infection rate of (A) R. typhina, (B) C album, (C) V. negundo, (D) R. chinensis and (E) A. truncatum. Means with the different letters are significantly different at P<0.05 in different treatments.
N deposition had significant effects on the aboveground biomass of C. album, V. negundo and native community, the SLA of C. album and A. truncatum, and the CHL of C. album, A. truncatum and native plant community (Table 1). The effects of AMF inoculation on all traits of both R. typhina and the native plants were insignificant (Table 1). We found significant interactive effects of N deposition and AMF inoculation on the CHL of R. typhina and the SLA of A. truncatum (Table 1).
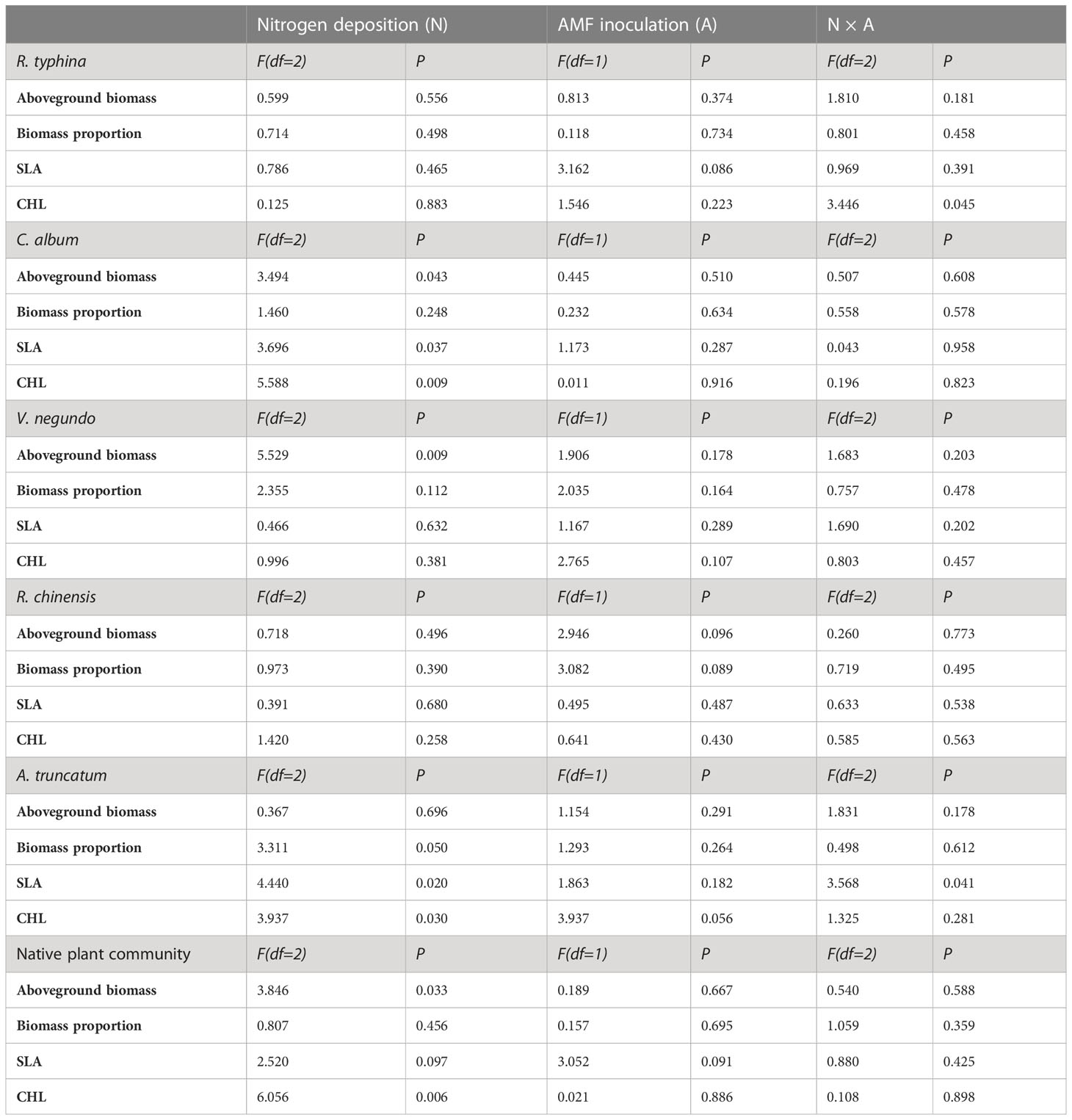
Table 1 F-values and significances of two-way ANOVA of the effects of nitrogen deposition and arbuscular mycorrhizal fungi (AMF) inoculation on the plant performance of R. typhina and native plant community.
Biomass
The aboveground biomass of C. album, V. negundo and native community significantly increased with the increase of N deposition level without AMF inoculation, while their aboveground biomass did not significantly vary with the increase of N deposition level with AMF inoculation (Figures 2B, C, F). No significant variations in aboveground biomass were detected for the other species (Figures 2A, D, E).
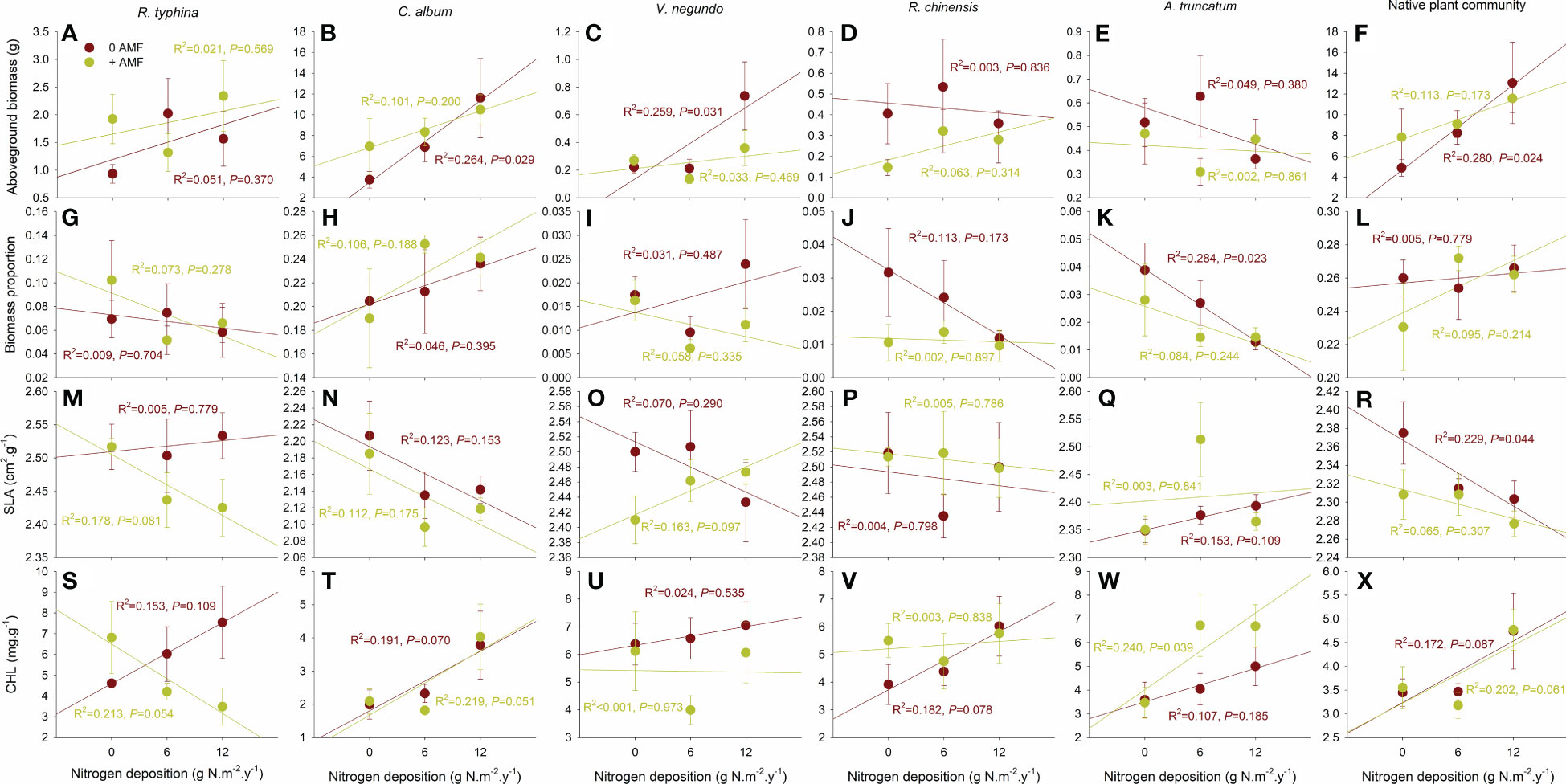
Figure 2 Correlations between nitrogen deposition level and aboveground biomass, biomass proportion, SLA and CHL for R. typhina (A, G, M, S), C. album (B, H, N, T), V. negundo (C, I, O, U), R. chinensis (D, J, P, V), A. truncatum (E, K, Q, W) and native plant community (F, L, R, X) without and with arbuscular mycorrhizal fungi (AMF) inoculation.
The biomass proportion of A. truncatum significantly decreased with the increase of nitrogen deposition level without AMF inoculation, while that did not significantly vary with the increase of nitrogen deposition level with AMF inoculation (Figure 2K). No significant variations in biomass proportion were shown for the other species (Figures 2G–J, L).
Leaf traits
The SLA of native community significantly decreased with the increase of nitrogen deposition level without AMF inoculation, while that did not significantly vary with the increase of nitrogen deposition level with AMF inoculation (Figure 2R). No significant variations in SLA were shown for the other species (Figure 2M–Q).
The CHL of A. truncatum did not significantly vary with the increase of nitrogen deposition level without AMF inoculation, while that significantly increased with the increase of nitrogen deposition level with AMF inoculation (Figure 2W). Despite that no significant variations in CHL were found for the other species (Figure 2T–V, X), a contrast variation trend of CHL for R. typhina appeared to be shown: an increase of CHL following the increase of N deposition level without AMF inoculation (P = 0.109) however a decrease of CHL following the increase of N deposition level with AMF inoculation (P = 0.054) (Figure 2S).
Discussion
Based on the evaluation of traits including biomass, biomass proportion, SLA and CHL, the present study showed that AMF inoculation had weak effects on both R. typhina and native plants under n deposition. Biomass accumulation usually represents the assimilatory power of plants and reflects the capacity of plants to colonize the resource niche (Yan et al., 2020). In accordance with the above perspective, biomass accumulation has been recognized to be positively correlated with the invasiveness of alien plants and the biotic resistance of native plant communities to invasion (Yuan et al., 2013; Liu et al., 2018; Guo et al., 2020; Jin et al., 2022; Li et al., 2022a; Li et al., 2022b; Wang et al., 2022; Xing et al., 2022). AMF inoculation impeded the increase of aboveground biomass for C. album, V. negundo and native plant community, and the decrease of biomass proportion for A. truncatum under nitrogen deposition, while no significant effects of AMF inoculation were found under remaining conditions. Considering invasiveness and invasibility, AMF inoculation did not enhance the performance of the exotic invader, R. typhina across different deposition levels, despite that a weakened resistance of native plant community with AMF inoculation. The plant–plant interaction, such as competition between the invader and native, and among the species within the native plant community was likely stable (Ma et al., 2017). A stability may exist for the community assembled by the alien invader and native plants since the community could “tolerate” the environmental perturbation from N deposition and AMF inoculation (Ghedini and Connell, 2016). Previous studies on the effects of environmental perturbation on the invasibility of native plant community showed two contrast paradigms including: 1) the invader up and the native down, in which the invasion process strengthens; and 2) the invader down and the native up, in which the biotic resistance strengthens (Liu et al., 2018; Zheng et al., 2020; Gao et al., 2021; Wang et al., 2021; Jin et al., 2022; Li et al., 2022b; Liu et al., 2022). However, the present study tends to show a balance between the alien invader and native plant community, especially considering the phenotypic maintenance of alien invader. Functional traits such as SLA and chlorophyll concentration are fundamentally associated with the resource use for instance the carbon gain and have been widely considered to be positively correlated with the photosynthetic efficiency and plant growth (Wang et al., 2017). And the evidence from these two traits also showed the balance between the alien invader and native plant community. The trait performance in SLA and chlorophyll concentration corresponds to biomass accumulation (a proxy for plant growth), since under most conditions the response of these two traits to nitrogen deposition insignificantly altered with AMF inoculation. The constant outcome of plant–plant interaction between the alien invader and native plant community may implicate a stable status of invasion dynamic. Despite that, the alien invader, R. typhina showed a disparate variation tendency for CHL following the increase of N deposition level under different AMF inoculation conditions, as N deposition increased the CHL without AMF inoculation while a contrast pattern was shown. Despite a lack of statistical significance, AMF inoculation was probably disadvantageous for the invasion of R. typhina into native plant community when facing nitrogen deposition, which needs further investigation.
Counterintuitively, N deposition and AMF inoculation, which have been certified to influence the resource availability for plants directly and indirectly, exerted weak impacts on the performances of the alien invader and native plants in the present study (Smith and Read, 2008; Wang et al., 2017; Wipf et al., 2019; Frew et al., 2020; Wang et al., 2022; Xing et al., 2022). Two probable explanations may be involved in this stability. The first explanation deals with the growth habits of woody plants. Previous studies on the invasibility of native plant communities commonly used fast-growing herbaceous plants as experimental materials (Liu et al., 2018; Jin et al., 2022; Li et al., 2022b). These herbaceous plants are more sensitive to resource fluctuation than woody plants (Rich et al., 2008). Since woody plants are slow-growing, the response of woody plants to resource fluctuation may be not prompt (Kozlowski et al., 1991). The second explanation relates to the role of AMF inoculation in the promotion of plant performance. Kiers et al. (2000) showed that the effects of AMF inoculation on plant performance are dependent on the host species. Hence, we speculate that the inoculation of AMF has weak effects on promoting the nutrient acquisition of the plants in the present study.
The present study provides a novel insight into the effects of environmental perturbation on the invasibility of native plant communities. In contrast to the prior studies with similar topics, we found that a neutral effect of environmental perturbation on the contrast between an alien woody invader and a native plant community dominated by woody plants. In summary, a constant pattern of invasion dynamic for the woody communities may exist comparing to the shifting pattern in the invasion dynamic for the herbaceous communities under environmental perturbation. We suggest that the future study on the effects of environmental perturbation on the invasion of woody invaders into native woody communities should consider temporal scale as a priority because of the slow growth of woody plants. Importantly, as alien invaders like R. typhina have strong allelopathic effects and may induce an interfering competitive effect on the native plants, the direct competition for resources is not the only form of plant–plant interactions between the alien invader and native plant community (Young et al., 2022). The structure of these plant–plant interactions should be probed in the mesocosm experiments of artificial plant communities. Although the present study implicates a neutral plant–plant interaction between the alien invader and native community, the interaction may convert mutually among competition, neutrality and facilitation with the change of environmental perturbation (Brooker, 2006). Further study should explore the threshold values of key ecological factors modifying the plant–plant interactions. By using this approach, a deeper insight on community invasibility can be perceived by the integration of plant–plant interactions and plant–environment interactions.
Data availability statement
The raw data supporting the conclusions of this article will be made available by the authors, without undue reservation.
Author contributions
XG and TW proposed the idea, designed the experiment, analyzed the data and wrote the manuscript. ML and SG revised the manuscript. SJ and LX set up the experiment, performed the harvest and collected the data. LY performed the visualization. All authors contributed to the article and approved the submitted version.
Funding
This study was financially supported by the Natural Science Foundation of China (32271588) and the Qingdao Agricultural University Doctoral Start-Up Fund (663/1121009).
Conflict of interest
The authors declare that the research was conducted in the absence of any commercial or financial relationships that could be construed as a potential conflict of interest.
Publisher’s note
All claims expressed in this article are solely those of the authors and do not necessarily represent those of their affiliated organizations, or those of the publisher, the editors and the reviewers. Any product that may be evaluated in this article, or claim that may be made by its manufacturer, is not guaranteed or endorsed by the publisher.
Supplementary material
The Supplementary Material for this article can be found online at: https://www.frontiersin.org/articles/10.3389/fevo.2023.1152213/full#supplementary-material
References
Borer E. T., Stevens C. J. (2022). Nitrogen deposition and climate: an integrated synthesis. Trends Ecol. Evol. 37, 541–552. doi: 10.1016/j.tree.2022.02.013
Brooker R. W. (2006). Plant–plant interactions and environmental change. New Phytol. 171, 271–284. doi: 10.1111/j.1469-8137.2006.01752.x
Bunn R. A., Ramsey P. W., Lekberg Y. (2015). Do native and invasive plants differ in their interactions with arbuscular mycorrhizal fungi? A meta-analysis. J. Ecol. 103, 1547–1556. doi: 10.1111/1365-2745.12456
Cavieres L. A. (2021). Facilitation and the invasibility of plant communities. J. Ecol. 109, 2019–2028. doi: 10.1111/1365-2745.13627
Cheng J. K., Yue M. F., Yang H. R., Chen B. M., Xing G. R. (2019). Do arbuscular mycorrhizal fungi help the native species Bidens biternata resist the invasion of Bidens alba? Plant Soil 444, 443–455. doi: 10.1007/s11104-019-04297-2
Curtis J. T., McIntosh R. P. (1951). An upland forest continuum in the prairie-forest border region of Wisconsin. Ecology 32, 476–496. doi: 10.2307/1931725
Davis M. A., Grime J. P., Thompson K. (2000). Fluctuating resources in plant communities: a general theory of invasibility. J. Ecol. 88, 528–534. doi: 10.1046/j.1365-2745.2000.00473.x
Dong L. J., Ma L. N., He W. M. (2021). Arbuscular mycorrhizal fungi help explain invasion success of Solidago canadensis. Appl. Soil Ecol. 157, 103763. doi: 10.1016/j.apsoil.2020.103763
Frew A., Powell J. R., Johnson S. N. (2020). Aboveground resource allocation in response to root herbivory as affected by the arbuscular mycorrhizal symbiosis. Plant Soil 447, 463–473. doi: 10.1007/s11104-019-04399-x
Gao F. L., He Q. S., Xie R. Q., Hou J. H., Shi C. L., Li J. M., et al. (2021). Interactive effects of nutrient availability, fluctuating supply, and plant parasitism on the post-invasion success of Bidens pilosa. Biol. Invasions 23, 3035–3046. doi: 10.1007/s10530-021-02555-y
Garnier E., Cortez J., Billes G., Navas M., Roumet C., Debussche M., et al. (2004). Plant functional markers capture ecosystem properties during secondary succession. Ecology 85, 2630–2637. doi: 10.1890/03-0799
Ghedini G., Connell S. D. (2016). Organismal homeostasis buffers the effects of abiotic change on community dynamics. Ecology 97, 2671–2679. doi: 10.1002/ecy.1488
Guo X., Xu Z. W., Li M. Y., Ren X. H., Liu J., Guo W. H. (2020). Increased soil moisture aggravated the competitive effects of the invasive tree Rhus typhina on the native tree Cotinus coggygria. BMC Ecol. 20, 17. doi: 10.1186/s12898-020-00284-9
Hayes F., Lloyd B., Mills G., Jones L., Dore A. J., Carnell E., et al. (2019). Impact of long-term nitrogen deposition on the response of dune grassland ecosystems to elevated summer ozone. Environ. Pollut. 253, 821–830. doi: 10.1016/j.envpol.2019.07.088
Isles P. D. F. (2020). The misuse of ratios in ecological stoichiometry. Ecology 101, e03153. doi: 10.1002/ecy.3153
Jansson J. K., Hofmockel K. S. (2020). Soil microbiomes and climate change. Nat. Rev. Microbiol. 18, 35–46. doi: 10.1038/s41579-019-0265-7
Jiang Y. N., Wang W. X., Xie Q. J., Liu N., Liu L. X., Wang D. P., et al. (2017). Plants transfer lipids to sustain colonization by mutualistic mycorrhizal and parasitic fungi. Science 356, 1172–1175. doi: 10.1126/science.aam9970
Jin H. F., Chang L., van Kleunen M., Liu Y. J. (2022). Soil mesofauna may buffer the negative effects of drought on alien plant invasion. J. Ecol. 110, 2332–2342. doi: 10.1111/1365-2745.13950
Kiaer L. P., Weisbach A. N., Weiner J., Gibson D. (2013). Root and shoot competition: a meta-analysis. J. Ecol. 101, 1298–1312. doi: 10.1111/1365-2745.12129
Kiers R. T., Lovelock C. E., Krueger E. L., Herre E. A. (2000). Differential effects of tropical arbuscular mycorrhizal fungal inocula on root colonization and tree seedling growth: implications for tropical forest diversity. Ecol. Lett. 3, 106–113. doi: 10.1046/j.1461-0248.2000.00126.x
Kozlowski T. T., Kramer P. J., Pallardy S. G. (1991). “How woody plants grow,” in The physiological ecology of woody plants. Eds. Kozlowski T. T., Kramer P. J., Pallardy S. G. (San Diego, California: Academic Press, Inc), 1–30.
Lamit L. J., Giovati A. S., Jo I., Frank D. A., Fridley J. D. (2022). Woody invaders are more highly colonized by arbuscular mycorrhizal fungi than congeneric native species. Am. J. Bot. 109, 655–663. doi: 10.1002/ajb2.1839
Li Y. J., Gao Y. Z., van Kleunen M., Liu Y. J. (2022b). Herbivory may mediate the effects of nutrients on the dominance of alien plants. Funct. Ecol. 36, 1292–1302. doi: 10.1111/1365-2435.14019
Li S. P., Jia P., Fan S. Y., Wu Y. T., Liu X., Meng Y. N., et al. (2022a). Functional traits explain the consistent resistance of biodiversity to plant invasion under nitrogen enrichment. Ecol. Lett. 25, 778–789. doi: 10.1111/ele.13951
Liu X. ,. S., Cai J. F., Liu L., Sun K., Jiang F., Shen Y. L., et al. (2022). Nitrogen addition frequency and propagule pressure influence Solidago canadensis invasion into native plant community. Ecosyst. Health Sust. 8, 2052763. doi: 10.1080/20964129.2022.2052763
Liu Y. J., Zhang X. Q., van Kleunen M. (2018). Increases and fluctuations in nutrient availability do not promote dominance of alien plants in synthetic communities of common natives. Funct. Ecol. 32, 2594–2604. doi: 10.1111/1365-2435.13199
Lopez B. E., Allen J. M., Dukes J. S., Bradley B. A. (2022). Global environmental changes more frequently offset than intensify detrimental effects of biological invasions. Proc. Natl. Acad. Sci. U.S.A. 119, e2117389119. doi: 10.1073/pnas.2117389119
Lü C. Q., Tian H. Q. (2007). Spatial and temporal patterns of nitrogen deposition in China: synthesis of observational data. J. Geophys. Res. 112, D22S05. doi: 10.1029/2006JD007990
Luo Y., Guo W., Yuan Y., Liu J., Du N., Wang R. (2014). Increased nitrogen deposition alleviated the competitive effects of the introduced invasive plant Robinia pseudoacacia on the native tree Quercus acutissima. Plant Soil 385, 63–75. doi: 10.1007/s11104-014-2227-1
Ma Z. Y., Liu H. Y., Mi Z. R., Zhang Z. H., Wang Y. H., Xu W., et al. (2017). Climate warming reduces the temporal stability of plant community biomass production. Nat. Commun. 8, 15378. doi: 10.1038/ncomms15378
Maron J. L., Marler M. (2008). Effects of native species diversity and resource additions on invader impact. Am. Nat. 172, S18–S33. doi: 10.1086/588303
Millennium Ecosystem Assessment (2005). Ecosystems and human well-being: biodiversity synthesis (Washington, DC: World Resources Institute).
Peng Y. F., Peng Z. P., Zeng X. T., Houx III, J. H. (2019). Effects of nitrogen-phosphorus imbalance on plant biomass production: a global perspective. Plant Soil 436, 245–252. doi: 10.1007/s11104-018-03927-5
Penuelas J., Janssens I. A., Ciais P., Obersteiner M., Sardans J. (2020). Anthropogenic global shifts in biospheric n and p concentrations and ratios and their impacts on biodiversity, ecosystem productivity, food security, and human health. Global Change Biol. 26, 1962–1985. doi: 10.1111/gcb.14981
Rich P. M., Breshears D. D., White A. B. (2008). Phenology of mixed woody–herbaceous ecosystems following extreme events: net and differential responses. Ecology 89, 342–352. doi: 10.1890/06-2137.1
Richardson D. M., Hui C., Nuñez M. A., Pauchard A. (2014). Tree invasions: patterns, processes, challenges and opportunities. Biol. Invasions 16, 473–481. doi: 10.1007/s10530-013-0606-9
Richardson D. M., Rejmánek M. (2011). Trees and shrubs as invasive alien species – a global review. Divers. Distrib. 17, 788–809. doi: 10.1111/j.1472-4642.2011.00782.x
Sun D. S., Yang X. P., Wang Y., Fan Y., Ding P. C., Song X. E., et al. (2022). Stronger mutualistic interactions with arbuscular mycorrhizal fungi help asteraceae invaders outcompete the phylogenetically related natives. New Phytol. 236, 1487–1496. doi: 10.1111/nph.18435
Valliere J. M., Irvine I. C., Santiago L., Allen E. B. (2017). High n, dry: experimental nitrogen deposition exacerbates native shrub loss and nonnative plant invasion during extreme drought. Global Change Biol. 23, 4333–4345. doi: 10.1111/gcb.13694
Vitousek P. M. (1992). Global environmental change: an introduction. Annu. Rev. Ecol. Evol. S. 23, 1–14. doi: 10.1146/annurev.es.23.110192.000245
Wang Z. X., He Z. S., He W. M. (2021). Nighttime climate warming enhances inhibitory effects of atmospheric nitrogen deposition on the success of invasive Solidago canadensis. Climatic Change 167, 20. doi: 10.1007/s10584-021-03175-0
Wang T., Hu J. T., Gao Y. Y., Yu D., Liu C. H. (2017). Disturbance, trait similarities, and trait advantages facilitate the invasion success of Alternanthera philoxeroides (Mart.) Griseb. CLEAN-Soil Air Water 45, 1600437. doi: 10.1002/clen.201600378
Wang T., Hu J. T., Wang R. Q., Liu C. H., Yu D. (2019). Trait convergence and niche differentiation of two exotic invasive free-floating plant species in China under shifted water nutrient stoichiometric regimes. Environ. Sci. Pollut. R. 26, 35779–35786. doi: 10.1007/s11356-019-06304-6
Wang G. M., Jiang G. M., Yu S. L., Li Y. H., Liu H. (2008). Invasion possibility and potential effects of Rhus typhina on Beijing municipality. J. Integr. Plant Biol. 50, 522–530. doi: 10.1111/j.1744-7909.2008.00660.x
Wang Q., Li M. Y., Eller F., Luo Y. J., Nong Y. L., Xing L. J., et al. (2022). Trait value and phenotypic integration contribute to the response of exotic Rhus typhina to heterogeneous nitrogen deposition: a comparison with native Rhus chinensis. Sci. Tot. Env. 844, 157199. doi: 10.1016/j.scitotenv.2022.157199
Wang C. Y., Liu J., Xiao H. G., Zhou J. W. (2016). Differences in leaf functional traits between Rhus typhina and native species. CLEAN-Soil Air Water 44, 1591–1597. doi: 10.1002/clen.201600144
Wang T., Zhu Y., Zhang Z. Y., Chi X. L., Huang X. Y., Zhang M. Y., et al. (2023). Pervasive native plant has the potential to resist the invasion of exotic species: a trait-based comparison. Hydrobiologia 850, 2015–2033. doi: 10.1007/s10750-023-05212-8
Wipf D., Krajinski F., van Tuinen D., Recorbet G., Courty P. (2019). Trading on the arbuscular mycorrhiza market: from arbuscules to common mycorrhizal networks. New Phytol. 223, 1127–1142. doi: 10.1111/nph.15775
Xing L. J., Li M. Y., Jiang S. Y., Li W., Guo S. X., Li H. M., et al. (2022). Comparison between the exotic Coreopsis grandiflora and native Dendranthema indicum across variable nitrogen deposition conditions. Acta Physiol. Plant 44, 82. doi: 10.1007/s11738-022-03417-3
Xu Z. L., Zhong S. S., Yu Y. L., Wang Y. Y., Cheng H. Y., Du D. L., et al. (2023). Rhus typhina L. triggered greater allelopathic effects than Koelreuteria paniculata Laxm under ammonium fertilization. Sci. Hortic-Amsterdam 309, 111703. doi: 10.1016/j.scienta.2022.111703
Yan X. B., Diez J., Huang K. L., Li S. P., Luo X., Xu X. Y., et al. (2020). Beyond resource limitation: an expanded test of the niche dimension hypothesis for multiple types of niche axes. Oecologia 193, 689–699. doi: 10.1007/s00442-020-04713-w
Young S. L., Kimball S., Novak S. J. (2022). “Invasion of plant communities,” in Global plant invasions. Eds. Clements D. R., Upadhyaya M. K., Joshi S., Shrestha A. (Cham: Springer), 29–51.
Yu G. R., Jia Y. L., He N. P., Zhu J. X., Chen Z., Wang Q. F., et al. (2019). Stabilization of atmospheric nitrogen deposition in China over the past decade. Nat. Geosci. 12, 424–429. doi: 10.1038/s41561-019-0352-4
Yuan Y. F., Guo W. H., Ding W. J., Du N., Luo Y. J., Liu J., et al. (2013). Competitive interaction between the exotic plant Rhus typhina L. and the native tree Quercus acutissima Carr. in Northern China under different soil N:P ratios. Plant Soil 372, 389–400. doi: 10.1007/s11104-013-1748-3
Zhang Y., Dore A. J., Liu X., Zhang F. (2011). Simulation of nitrogen deposition in the North China Plain by the FRAME model. Biogeosciences 8, 3319–3329. doi: 10.5194/bg-8-3319-2011
Zhang Z. J., van Kleunen M. (2019). Common alien plants are more competitive than rare natives but not than common natives. Ecol. Lett. 22, 1378–1386. doi: 10.1111/ele.13320
Zhao X. H., Wang T., Li Y. W., Li M. Y., Huang X. R. (2023). The alien invader, Rhus typhina l., outperforms its native competitor in the scenario of nitrogen deposition and arbuscular mycorrhizal fungi (AMF) inoculation. Sustainability 15, 6082. doi: 10.3390/su15076082
Keywords: global environmental change, arbuscular mycorrhizal fungi, invasibility, woody invader, native plant community, plant–plant interactions
Citation: Guo X, Li M, Jiang S, Yang L, Guo S, Xing L and Wang T (2023) Arbuscular mycorrhizal fungi inoculation exerts weak effects on species- and community-level growth traits for invading or native plants under nitrogen deposition. Front. Ecol. Evol. 11:1152213. doi: 10.3389/fevo.2023.1152213
Received: 27 January 2023; Accepted: 04 July 2023;
Published: 17 July 2023.
Edited by:
Deliang Kong, Henan Agricultural University, ChinaReviewed by:
Amber Churchill, University of Minnesota, United StatesYuping Hou, Ludong University, China
Copyright © 2023 Guo, Li, Jiang, Yang, Guo, Xing and Wang. This is an open-access article distributed under the terms of the Creative Commons Attribution License (CC BY). The use, distribution or reproduction in other forums is permitted, provided the original author(s) and the copyright owner(s) are credited and that the original publication in this journal is cited, in accordance with accepted academic practice. No use, distribution or reproduction is permitted which does not comply with these terms.
*Correspondence: Tong Wang, ecotone@qau.edu.cn