Recent advances in the effective removal of hazardous pollutants from wastewater by using nanomaterials—A review
- 1School of Sciences, P. P. Savani University, Surat, Gujarat, India
- 2Department of Chemistry (Applied Sciences), Institute of engineering & Technology, NIMS University, Jaipur, Rajasthan, India
- 3Department of Chemistry, School of Liberal Arts and Sciences (SLAS), Mody University of Science and Technology, Lakshmangarh, Rajasthan, India
- 4Department of Chemistry, Institute of Science and Research, IPS Academy, Indore, Madhya Pradesh, India
- 5Department of Chemistry, Navyug Kanya Mahavidyalaya, University of Lucknow, Lucknow, Uttar Pradesh, India
- 6Department of Veterinary Clinical Sciences, College of Veterinary Medicine, Iowa State University, Ames, IA, United States
- 7Department of Life Sciences, Hemchandracharya North Gujarat University, Patan, Gujarat, India
Environmental nanotechnology has developed rapidly over the past few decades due to the fast advancement of nanotechnology and nanomaterials (NMs). Due to their nanoscale size, NMs are receiving immense attention in research and development worldwide. Their nano size has led to better catalysis, high reactivity, and high adsorption capacity. In wastewater treatment, nanotechnology has significant potential to improve the performance and efficiency of water decontamination; more effectively, it provides a sustainable way to keep water supplies safe. Numerous studies have found that removing harmful components from wastewater by employing nanoparticles in conjunction with various treatment methods is effective. The purpose of the current investigation is to conduct a review of the envisioned applications of various NMs in the treatment of wastewater. These NMs include carbonaceous NMs, metal-containing nanoparticles, and nanocomposites, all of which will be reviewed and highlighted in depth.
1 Introduction
Nowadays, the scarcity of water has become a large-scale problem for everybody. Clean water is a basic necessity for various purposes, including domestic, agricultural, industrial, and energy needs, particularly in developing countries where the population is rising (He et al., 2021; Yadav et al., 2023a). Every year a huge amount of fresh water is contaminated by various water pollutants, ultimately making the water unfit for drinking (Amari et al., 2023). The major pollutants of water are dyes, heavy metals, pesticides, microorganisms, hydrocarbons, and other toxic substances which challenge a potential threat to aquatic and living organisms (Nazari et al., 2021; Afolalu et al., 2022; Zhou et al., 2022a). Among heavy metals, the most common ones are mercury, arsenic, copper, nickel, zinc, etc., In addition, it has been suggested that heavy metals like zinc and mercury can alter protein structure and result in cancer (Saeed and Shaker, 2008; Witkowska et al., 2021). Dyes discharged from the textile industries, etc., lead to water pollution and disturb aquatic life as dyes prevent the penetration of sunlight to the deeper parts of the water bodies (Islam et al., 2023). Dyes polluted water may cause skin disorders and, in the long term, may cause cancer in living beings. Another major pollutant of water is pesticides (organophosphate, carbamate, organochlorine, pyrethroids, etc.) which come mainly from irrigation and agriculture (Ajiboye et al., 2022; Mishra et al., 2023). The agricultural fields introduced with pesticides leached with the rainwater and other water activities, ultimately reaching the freshwater bodies (Malla et al., 2021). These pesticides may accumulate in aquatic animals, which on consumption by humans, may lead to biomagnification (Ali et al., 2020; Gupta and Gupta, 2020). The consumption of pesticide-contaminated water in the long term may lead to numerous health-related disorders (Rajput et al., 2021; Tang et al., 2021). Pathogenic microorganisms are another major source of water pollution which mainly causes food and waterborne diseases (Kumar et al., 2021).
These effluents cause problems, including metal poisoning, irritations, and pathogenic infections in humans and animals (Briffa et al., 2020; Gaur et al., 2021). Good quality water is essential to sustain human wellbeing, livelihoods, and a healthy environment for sustainable development. As per the March 2020 WHO report, only 74% of the world’s population (5.8 billion people) has access to safe water, while around two billion people use water contaminated with feces. In furtherance of this discussion, it appears that approximately 50% of the world’s population will encounter water scarcity by 2025. In the past, the management of wastewater posed significant challenges. However, contemporary practices have evolved to include recycling, resulting in both wastewater treatment and a renewable energy source.
Currently, the majority of the investigations emphasize a particular method for the remediation of heavy metal ions, including electrocoagulation (EC), photocatalysis utilizing synthetic and natural adsorbents, the use of magnetic fields, advanced oxidation process (AOP), adsorption, membrane techniques, etc. (Singh et al., 2023a). Moreover, various nanomaterials (NMs) are utilized as nano-adsorbents, nano-catalysts, and nano-membranes for the treatment of wastewater effluents. Also, activated carbon nanotubes (CNTs), including both multi-walled and single-walled surfaces functionalized with decorated with zero-valent Ni NPs, are employed for the adsorption of heavy metals like (As, Cd, and Pb) from wastewater. Sagadevan et al. (2022) reported the titanium dioxide (TiO2) based photocatalytic remediation of dyes and heavy metals from wastewater, while Aragaw and co-workers developed biomass-based adsorbents for the remediation of dyes from wastewater. Interestingly, Burk et al. (2020) reported Chitosan-coated gasifier biochar for the remediation of Cd (II) and Cu (II) from aqueous solutions (Aragaw and Bogale, 2021; Sagadevan et al., 2022).
Different treatment techniques are applied to remove toxic contaminants from wastewater, including chemical, biological, and ion exchange techniques, adsorption, and photocatalysis (Titchou et al., 2021; Ahmed et al., 2022; Yadav et al., 2023b). These treatment methods employed aim to enhance water quality; however, certain limitations are associated with some of these techniques. For instance, chemical methods often demand the use of a substantial quantity of chemicals, necessitate pH monitoring, result in sludge formation, and generate secondary pollutants due to excessive chemical usage (Bijekar et al., 2022). Also, adsorption techniques produce optional toxins. However, photocatalysis is a method that produces reactive chemical species that convert toxic pollutants into non-toxic byproducts and is sustainable, environmentally friendly, and clean (Huang et al., 2022). Photocatalysis is a rapidly developing technology attracting the attention of investigators due to its low cost and high efficiency in water decontamination compared to other methods (Khan, 2021).
Other promising techniques include membrane filtration and AOPs (Titchou et al., 2021). Membrane technology enables the effective separation of dyestuffs and dyeing auxiliaries, which concurrently mitigate the hydrolyzed color and biochemical oxygen demand/chemical oxygen demand of wastewater. These processes are typically used to treat effluent-reactive dye baths, which have the potential to reduce waste volume and recover salt at the same time. The utilization of the membrane filtration technique offers numerous advantages, including its expeditious nature and minimal spatial requirements (Asif and Zhang, 2021; Bhol et al., 2021).
AOPs are a newer, more powerful, and promising set of techniques developed and used to treat dye-contaminated effluents. The AOP technique has garnered considerable attention from the scientific community due to its user-friendly nature and its ability to generate substantially reduced residuals compared to conventional methodologies. AOPs exhibit superior performance compared to all currently available methodologies, albeit at a significantly higher cost (Ma et al., 2021a; Priyadarshini et al., 2022).
Chemical precipitation involves many disadvantages, like the production of a high amount of sludge, toxic by-products, time-consuming processes, and slow aggregation and settling of metal ions precipitate (Saleh et al., 2022). The cost of the regeneration process in the adsorption technique is high and may lead to adsorbent loss and its’ effective performance. The frequent regeneration of ion-exchange resin in ion-exchange techniques leads to secondary pollution in the form of chemical reagents. Photocatalysis is mostly applicable for sludges and effluents, and the photo Fenton oxidation technique produces a large amount of iron-containing sludge (Al-Asheh and Aidan, 2020). Also, biological treatments are highly selective, toxic, sensitive to microorganisms, and require a large space for the bioreactors. Membrane processes (reverse osmosis, ultrafiltration, and nanofiltration) suffer from higher investment costs, maintenance, and operations. Membrane fouling high-pressure requirements for reverse osmosis are major disadvantages of this technique. AOPs outperform all existing ones but are much more expensive (Barakat, 2011; Qasem et al., 2021; Saleh et al., 2022).
Building upon the methodologies above, the field of nanoscale science is employed for the purposes of imaging, measuring, and modeling at its specific length scale. This utilization proves to be advantageous in the context of pollutant removal due to its recyclability, cost-effectiveness, and high efficiency. Recent research has indicated that there has been a notable increase in the industrial influence of nanotechnology applications (Puri et al., 2021).
Nanotechnology encompasses manipulating and studying matter at the atomic and molecular levels, focusing on dimensions approximately one billionth of a meter in scale (1 × 10−9 m = 1 nm) (Puri et al., 2021). A nanoparticle can generally be any size between 1 and 100 nm (Aniculaesei et al., 2019). Metallic nanoparticles (NPs) differ from bulk metals in their physical and chemical characteristics (e.g., lower melting points, higher specific surface areas, specific optical properties, specific mechanical strengths, and specific magnetizations), and these characteristics may be useful in a variety of industrial applications. Metals, metal oxides, polymers, and dendrimers are just a few of the many components that can be used to create these particles. Due to their distinctive features resulting from their small size and high surface area-to-volume ratio (SVR), synthetic NPs are engaged in numerous applications, including electronics, energy, medicine, and catalysis (Singh et al., 2022b). Several methods, such as chemical approaches (sol-gel, co-precipitation, etc.), physical vapor deposition (PVD), and material synthesis with template assistance, can be used to create synthetic NPs. Materials can suddenly display radically different properties when scaled down to the nanoscale from what they do at the macroscale (Saleh et al., 2022). For instance, opaque compounds can become transparent (like copper), inert substances can act as catalysts (like platinum), stable substances can catch fire (like aluminum), solids can convert into liquids at normal temperature (like gold), and insulators can act as conductors (like silicon) (Horikoshi and Serpone, 2013; Puri et al., 2021).
Due to their nanoscale size (˜100 nm), NMs mechanically and electrically show a different behavior, and some of their optical and magnetic properties also differ from conventional materials (Alshammari et al., 2020; Modi et al., 2022). In the last past decades, many researchers are devoted to NMs preparation and also optimize them for information processing, machine learning (Jia et al., 2021), remote sensing (Altug et al., 2022; Bharadwaj et al., 2022), biomedical (Singh et al., 2020; Materón et al., 2021; Bagur et al., 2022), defense area, textile (Kabir et al., 2020), agriculture (Khan et al., 2023) and food industries (Modi et al., 2023b), environmental cleaning, etc (Baig et al., 2021). Nanotechnology is extensively being explored as a potential alternative in wastewater treatments like detoxication of water, desalination, etc.
The authors searched keywords, nanoparticles, nanomaterials, and wastewater treatment, on science direct.com by keeping the year limit “2018 to 2023” and found about 17,573 articles till 14 June 2023, out of which 3192 articles were published in 2023, 4775 in 2022, 3777 in 2021, 2601 in 2020, 1923 in 2019 and 1305 in 2018. Moreover, out of these 17,573 articles, 10,167 were research articles, 3,870 were review articles, 2,51 were book chapters, 269 were short communication, 98 were encyclopedias, 50 were conference abstracts, 30 were editorials, 10 were minireviews, 12 were case reports, 8 were discussion, data articles, and news were 5, correspondence was 2, practical guidelines and video articles were one each and rest 504 were others. The above investigation suggests that nanomaterials-based wastewater treatment is one of the latest topics among the scientific community around the whole globe, which is evidenced by the continuous and drastic increase in the articles every year from 2018 to 2023. Furthermore, the prevalence of research articles in this field suggests that further investigations are required to address the issue of water pollutants. So, the authors here tried to bridge the gap by providing the state-of-the-art in the field of nanomaterial-based wastewater treatment.
In this review, the authors have introduced the diverse categories of NMs and underscored their distinct properties that can be harnessed for addressing various pollutants in wastewater. The authors highlighted the significance of various NMs in the process of remediating dyes, heavy metals, pathogenic microorganisms, pesticides, and other substances. In this study, the authors have examined different categories of nanomaterials and their respective characteristics, which are employed in the process of sewer water reclamation.
2 Different classes of nanomaterials
Nanomaterials can be artificially synthesized in laboratory settings by manipulating different parameters to meet specific requirements. Additionally, NMs can also occur naturally as a result of various natural processes and activities. So, based on their origin, a nanomaterial could be classified into two categories: natural NMs, and laboratory-synthesized NMs (Das et al., 2020; Baig et al., 2021). Moreover, NMs could also be categorized based on their shapes, briefly discussed below. Figure 1 shows the major types of NMs based on their origin source, materials, and dimensions.
2.1 Natural nanomaterial
Natural NMs can be defined as substances that are formed through biogeochemical processes in a natural manner, without any contribution from human activities. These are intrinsic and present in natural bodies, e.g., viruses (capsid) and bone substances (Amin et al., 2014). Moreover, there are also formed by various natural activities, like clay, etc.
2.2 Laboratory-manufactured NMs
Laboratory-manufactured NMs refer to NMs that are artificially synthesized through the application of various methods. The entities above are further categorized into four distinct classes:
2.2.1 Carbon-based NMs
Carbon is present in these NMs, which are mainly of three types, i.e., which are hollow, ellipsoids (fullerenes), or tubes (CNTs). A team led by Sheron reported many applications of these NPs (single, double, and multi-walled nanotubes), like enhanced movies and coatings, many lighter materials, gadgets, and wastewater treatment. The utilization of graphene and carbon nanotubes (CNTs) in industries is attributed to their exceptional characteristics, including their high mechanical strength and lightweight nature (Fritea et al., 2021; Sheoran et al., 2022).
2.2.2 Metal-based NMs
This class comprises metal oxides (Al2O3, TiO2) (Bousiakou et al., 2022), nano-sized gold, nano-sized silver (Bagur et al., 2022; Nadaf et al., 2022; Van Thuan et al., 2022), etc. Semiconductor quantum dots are crystals at the nanometer scale that possess distinctive photophysical characteristics, including optical properties that vary with size, high fluorescence quantum yields, and remarkable resistance to photobleaching (Villalva et al., 2021). The length of quantum dabs varies with their optical properties (Shah et al., 2015; Chopra et al., 2022).
2.2.3 Dendrimers
Dendrimer has a variety of chain closes on their surface, which is used to perform particular blended capacities. This property is necessary for catalysis. Similarly, 3-D dendrimers include inside depressions into which different atoms could be located, and they might be valuable for drug transportation, e.g., nano-sized polymers (Kaurav et al., 2023). A typical diagram of a dendrimer is shown in Figure 2.
2.2.4 Composites
These materials are used for combining NPs, which takes place with other NPs as well as large-sized materials. Mixing NMs with any metal, mass materials, and polymer can give rise to these composites (Gadore and Ahmaruzzaman, 2021; Levofloxacin et al., 2022; Zsirka et al., 2022).
2.3 Categorization of nanomaterial based on the dimension
The physical properties of some systems changed due to the spatial reduction of the nanoparticle structure. Based on dimensions, NMs (Figure 3) are classified as follows:
Zero dimension structures (0-D): System limited to three dimensions. All the dimensions are estimated within the nanoscale (less than 100 nm). It includes Ag/Au NPs, nanograins, nanoporous silicons, nanorings, and fullerene (Alam et al., 2021).
One dimension structures (1-D): System confined in two dimensions with mm long. It contains nanorods, nanotubes, and nanowires of metal oxides.
Two-dimension structures (2-D): System limited to one dimension. It includes graphene, plate-like shapes, nanofilms, and nanolayers.
Three-dimension structures (3-D): The system is not confined in any dimension. It includes dispersions of NPs, nanowires, nanotubes multi-nanolayers, and bulk powders (Jagadeesh et al., 2017; Ba-Abbad et al., 2022; Yadav et al., 2023b).
3 Properties of nanoparticles
At nanoscale bulk, the properties of materials change drastically in comparison to their bulk counterparts. In addition to high SVR at the nanoscale (Jin and Higaki, 2021). Nanoparticles exhibit various additional characteristics, including alterations in magnetic, optical, electrical and electronic, thermal, and surface properties (Sajid and Płotka-Wasylka, 2020). All these properties are exploited in medicine, drug delivery, and environmental cleanup, which are discussed below in detail. Figure 4 shows the various types of properties that change at the nanoscale.
3.1 Magnetic properties
The higher surface area of NPs makes them unique and attractive. Magnetic NPs are utilized for cooling purposes, visualization, bioprocessing, higher cache memory materials, magnetic storage device, magnetic printing, etc. Giant magnetoresistance is a nanoscale multilayer containing ferromagnet (iron, cobalt, nickel) and non-attractive support materials (chromium, copper) which are used in data storage in memory (Shirsath and Shirivastava, 2015; Jefremovas et al., 2021).
3.2 Optical properties
The energies of orbitals (HOMO and LUMO) are mostly impacted by the nano size of the electronic structure (Sajid and Płotka-Wasylka, 2020). Due to these electrons, optical production and adsorption happen. The optical properties of many metals and semiconductors are changed extensively. The colloidal tension of Au NPs has dark red shading, which becomes dark yellow with an increase in particle size (Table 1) (Gnanamoorthy et al., 2020; Murthy et al., 2020; Zhu et al., 2020; Khan, 2021).
3.3 Electrical and electronic properties
The influence of size on electrical properties is a significant factor governed by scattering, electronic effects, and alterations in microstructure. When the material’s dimension increases, it causes a reduction of defects, which results in a decrease in resistivity and an increase in conductivity. Decrease of dimension below a critical size, i.e., below De Broglie wavelength, results in a change of electronic structure, which takes place due to the widening of the bandgap and reduced electrical conductivity (Liu et al., 2021b).
3.4 Thermal properties
The exceptional properties of NPs include special heat, thermal conductivity (TC), and thermoelectricity (Almuallim et al., 2022; He et al., 2022). For instance, CNTs have a very strong TC, i.e., twice that of diamonds, and therefore act as great conductors of heat (Kumanek and Janas, 2019). Phonons are the primary means of determining thermal conductive properties and specific temperatures for nanotubes (Qian et al., 2021). Metal NPs have high TC compared to most liquids in solids, and the TC is greatly enhanced in nano liquids. For instance, at room temperature, the TC of Cu is approximately 700 folds that of H2O and 3000 times that of motor oil (Czaplicka et al., 2021; Zhou et al., 2022b). Aluminum oxide exhibits a higher TC when compared to water. Hence, it can be inferred that nanofluids exhibit a higher thermal conductivity than fluids that incorporate fine particles and conventional heat transfer fluids (Coccia et al., 2021). This phenomenon can be attributed to the positive correlation between surface area and heat transfer efficiency. Table-2 shows the TC of a few NMs.
3.5 Surface properties
Surface properties like surface energy, particle-particle interaction, and surface modification primarily determine the agglomeration state of the particles and, therefore, their effective size, especially under physiological conditions (Coccia et al., 2021; Urian et al., 2021). Thus, the biological identity of a nanomaterial is clearly influenced by differentiating surface properties. This behavior is particularly relevant for biomedical applications. NPs possess quantum properties due to their very high ratio of atoms on the surface in comparison to the interior of the particle. Consequently, NPs are subject to distinct laws that differ from those governing larger matters. For example, gravitational forces do not exert influence on them; rather, they are governed by forces such as Van der Waals interactions (Bantz et al., 2014).
Activated carbons do not fulfill the criteria established in the different definitions of NMs provided by the existing regulations. Some of the Regulations and Scientific guides that have been evaluated are:
European Commission Recommendation for the definition of Nanomaterials, 2011/696/EU. It was intended to be applied as an overarching framework with regard to other EU regulations (Benko, 2017).
NB: Activated Carbons have been cited by ECHA as an example of a substance that might be interpreted as a nanomaterial based on the VSSA criteria. According to the literature, activated carbons possess a high surface area, although they do not fall under the category of NMs. Rather, they exhibit highly porous structures (Heidarinejad et al., 2020; Rao et al., 2021).
4 Positive and negative aspects of nanotechnology and nanomaterials
4.1 Positive aspects of nanotechnology and nanomaterials
The majority of the technological goods we use now are made with nanotechnology. Nobody could have expected that a gadget with thousands of memory cells would be that small. The complex circuitry of the chip has achieved its objective by making it portable, enabling users to carry any electronic device from one location to another. We no longer need supercomputers to do easy mathematical computations. Instead, we can do even more complicated calculations on smartphones (Thiruvengadam et al., 2018; Nile et al., 2020).
Nanotechnology has substantially enhanced medical research, thereby providing a valuable contribution to the healthcare sector (Anjum et al., 2021). The illness can now be easily detected, and treatment options are widely available. The medical profession has invented several drugs and medical equipment, such as nanorobots, to treat incurable medical conditions such as cancer by completely utilizing nanotechnology (Haleem et al., 2023). As a result, nanotechnology is advantageous to the healthcare sector. Nanotechnology is often used to detect and treat hidden disorders. Any critically ill person can now be easily accessed and diagnosed using a range of technologies that were formerly huge and immovable (Curvino et al., 2021).
4.1.1 Benefits of production
Modern manufacturing requires nanoproducts such as nanotubes, NPs, nanobatteries, and so on that are more resilient, powerful, and lightweight than comparable products created without the use of nanotechnology. Hence, due to nanotechnology, the environment for manufacturing has changed and has become much better for them (Hansen et al., 2020).
4.1.2 Energy creation
Nanotechnology has considerably aided in the field of energy generation. Batteries, cells, and various other energy-efficient storage devices have become commonplace. All of these have been demonstrated to be energy-saving devices that have enhanced people’s lives (Pomerantseva et al., 2019; Manickam et al., 2021).
Due to the significant advancements facilitated by nanotechnology have greatly enhanced the potential to effectively address diseases. A wide array of tools and instruments have been employed in managing and mitigating diverse chronic diseases and ailments that currently lack a definitive cure. The diagnosis of the illness can be facilitated through the utilization of nanotechnology. After a diagnosis, treating the medical condition and helping the patient recover quickly is much easier.
4.2 Negative aspects of nanotechnology and nanomaterials
4.2.1 Negative environmental impact
The progression of nanotechnology has led to a rise in pollution, primarily related to the generation of NPs while manufacturing diverse pharmaceuticals, atomic weaponry, and other commodities. As a consequence, nanotechnology has a substantial environmental impact. In addition to human beings, the animals inhabiting these areas have been affected by various diseases (Del Prado-Audelo et al., 2021; Phillips, 2021).
4.2.2 A rise in unemployment is possible
The advancement of science and technology has significantly reduced the demand for human labour. As a consequence, a significant number of individuals have relinquished their employment positions due to technological advancements replacing their roles. Engineering nanotechnology has led to enhanced machine functionalities and the reduction of labour-intensive positions, particularly in the field of chemistry (Ma et al., 2021b; Pokrajac et al., 2021).
4.2.3 Accessible dangerous weapons
Numerous weapons generated through nanotechnology exhibit deleterious properties and are vulnerable to misuse by humans. In today’s world, countries employ a diverse array of armaments to enhance their military capabilities. In the contemporary era, a nation possesses the capacity to construct and utilize weaponry such as atomic bombs with relative ease, thereby enabling the destruction of its adversaries (Khan et al., 2019).
4.2.4 Expensiveness
Nanotechnology, while advantageous in the fields of medicine, engineering, and material sciences, incurs significant expenses due to elevated operating and raw material expenditures. Consequently, the acquisition of the technology generally proves to be prohibitively costly for individuals of average means (Ray and Bandyopadhyay, 2021).
4.2.5 Nanotoxicity associated with the nanoparticles
The entry of these NPs into the ecosystem can occur through various pathways such as air, water, and soil, potentially resulting in nano-toxicity. Furthermore, as a result of its small size, it has the potential to permeate the dermal pores of individuals and contribute to the occurrence of metal-associated diseases, such as those associated with the utilization of zinc oxide (ZnO), titanium dioxide (TiO2), and silver (Ag) in cosmetic and toothpaste products.
5 Applications of nanomaterials for wastewater treatment
Numerous contaminants in water waste are detected and removed by applying nanotechnology. Non-biodegradable heavy metals are very toxic and adversely affect the lives of animals, plants, and living organisms, which become a scary situation for the environment (Yadav et al., 2023b). This problem can be solved by using NPs in the form of metal oxides (Ti, Zn), membranes (ceramic, polymer, nanowire, polymer), CNTs, nanopowder, etc. Water quality can be improved by different methods available like photocatalysis, electrochemical oxidation, nanofiltration, and adsorption methods which utilize the above-said materials (Yadav et al., 2022a).
NPs play a different role in the removal of toxic ions through adsorption, and chemical or photochemical oxidation processes, which is necessary for contaminants’ destruction (Isawi, 2020). Another important role of NMs is as functional materials such as carbonaceous NMs, nano adsorbents, nanofibers, nano clays (Biswas et al., 2020), zeolites (Murukutti and Jena, 2022), and dendrites. Various NMs are used for the treatment and purification of water (Figure 5) (Singh et al., 2022a).
5.1 Carbonaceous nanomaterials for wastewater treatment
In the current decade, dyes and heavy metals are removed by using various kinds of carbon-containing NMs due to their non-toxicity, structure, abundance, high surface area, porosity, and good sorption limits (Fritea et al., 2021; Gacem et al., 2022).
5.1.1 Activated carbon
Agricultural wastes coal, wood, and coconut shells are used as carbon-based precursors for the synthesis of activated carbon, which possesses high porosity and high surface area and is used as sorbents (Igwegbe et al., 2021; Yilmaz et al., 2022). Machado and their group used coconut tree sawdust and prepared activated carbon, which was then utilized for Cr (VI) remediation. Arcibar-Orozco et al. studied the phosphate effect in forced hydrolysis of ferric chloride on modified granular activated carbon (Saleem et al., 2019; Rajendran et al., 2021).
5.1.2 Graphene-based nanomaterials
Graphene forms a graphite structure in a two-dimensional honeycomb pattern that shows tremendous thermal and electrical conductivity. Graphene oxide (GO), which consists of hydroxyl, epoxy, and carbonyl groups, is obtained by monolayer graphene with oxidative form. Zhu et al. (2016) elucidated five potential interactions, namely, hydrogen bonding, π-π bonding, the hydrophobic effect, covalent bonding, and electrostatic interactions, that contribute to the process of adsorption (Zhu et al., 2016). Xu and Wang (2017) reported graphene-based material for wastewater treatment, which has a large surface area and oxygen in large quantities. Avouris and Dimitrakopoulos compared reduced graphene oxide (rGO) and graphene and found that functional group modification of rGO improved its’ imperfectness and conduction (Xu and Wang, 2017). The preparation of both of these oxides is shown in Figure 6.
For the remediation of contaminants such as heavy metals of lead, zinc, copper, cadmium, mercury, and arsenic, graphene-based materials act as good adsorbents. The utilization of two effective methods, namely, surface modification, and hybridization, enhances the working efficiency and reusing capacity of these materials. These substances have proven to be highly effective in the process of water decontamination, efficiently eliminating a wide range of pollutants (Mehdizadeh et al., 2014; Yadav and Fulekar, 2018; Irannajad and Kamran Haghighi, 2021). Although, their high cost is one of the main limitations in their application for environmental protection. Contaminants (metals and dyes) are removed by these materials due to their adsorption capacity, and for organic pollutants, removal by graphene, GO, rGO, and modified graphene is utilized, as shown in Table 3.
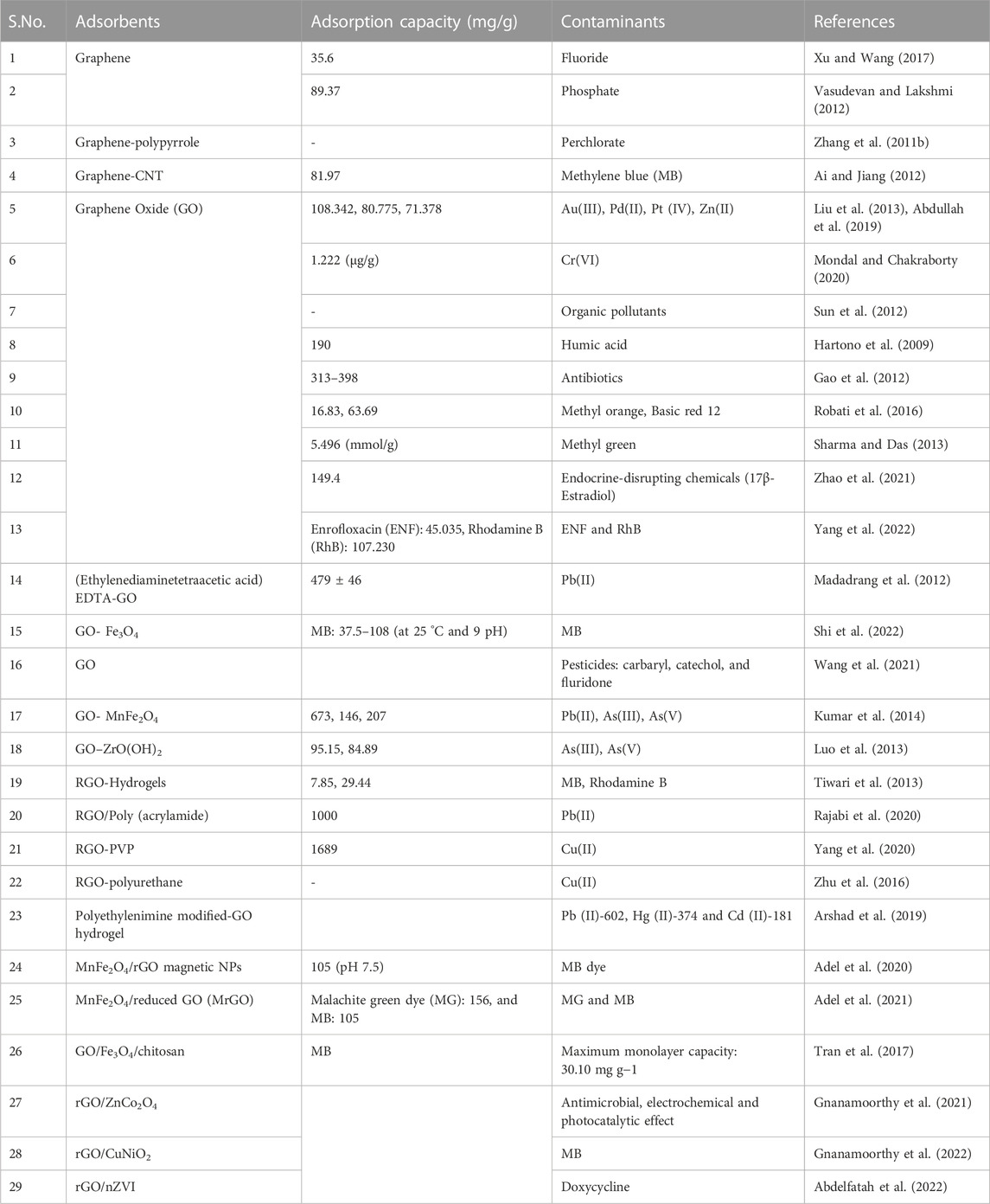
TABLE 3. Applications of graphene, GO, rGO, and modified graphene as adsorbents for contaminants removal from wastewater.
5.1.3 Carbon nanotubes (CNTs)
Carbon nanotubes possess new exceptional structural, mechanical, electrical, and magnetic properties, which make them unique in nanoelectronics. CNTs are mainly composed of carbon and exhibit stability, low reactivity and act as strong antioxidants. Their primary examples are CNTs, nanodiamonds, Fullerenes/Buckyballs (C60, C20, C70), and nanowires. These occur in different varieties like ellipsoids, nanowires, buckyballs, tubes (nanotubes), and nanodiamonds (Lin et al., 2018; Balarak et al., 2021). CNTs are used for wastewater management due to their easy conversion, large adsorption capacity, cylindrical hollow structure, high ratio aspect, and hydrophobic wall surfaces (Gacem et al., 2022). A team led by Rajabi et al. (2017) highlighted the utilization of multi-walled CNTs for the aqueous removal of methylene-based dyes like methylene red and MB. Table 4 displays the comparative results of CNTs and their adsorption capacity by which heavy metal ions get removed.
5.2 Metal-containing nanoparticles
Several nano-sized metals and metal oxides are used widely for the remediation of pollutants from water waste due to their higher efficiency and economical cost. These metal oxide NPs mainly include nano zero-valent iron (nZVI), Fe2O3, Al2O3, MnO, TiO2, MgO, CeO2, ZnO, and TiO2 (Naseem and Durrani, 2021; Aragaw and Ayalew, 2023; Singh et al., 2023b; Inamdar et al., 2023). Applications of all these NPs for the remediation of wastewater contaminants are shown in Table 5.
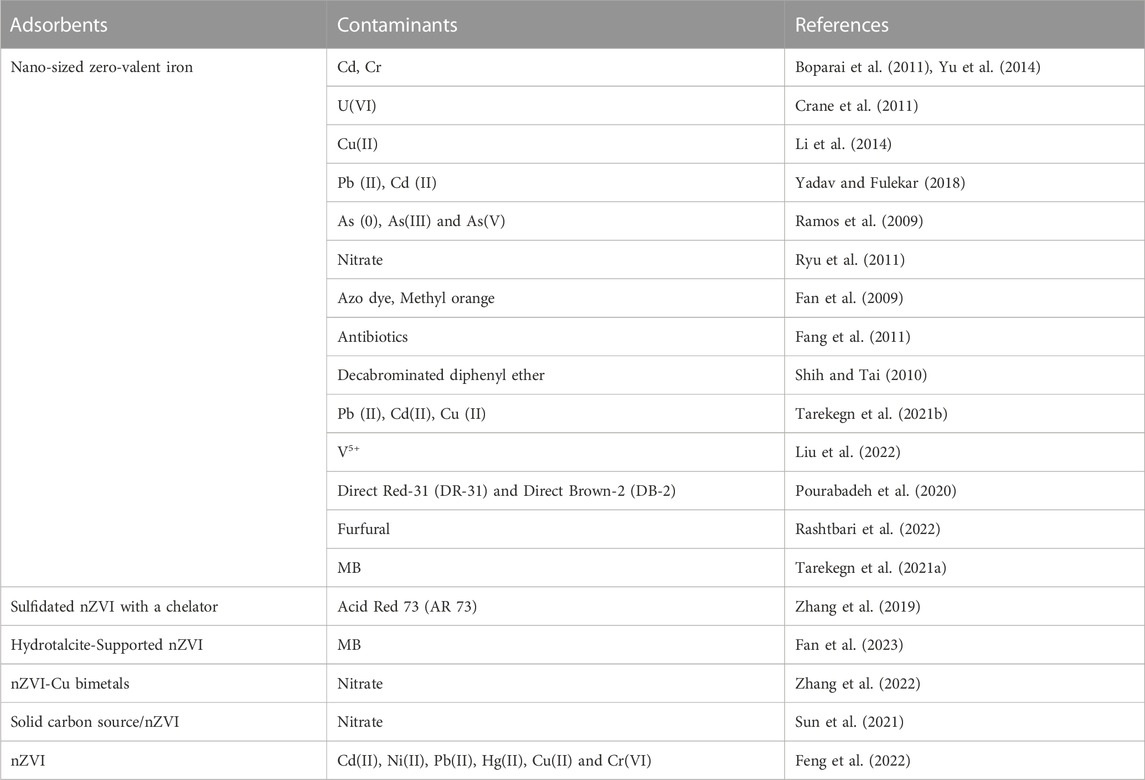
TABLE 5. Remediation of heavy metals and other pollutants using nano-sized zero-valent iron from wastewater.
5.2.1 Nanosized iron
Nanosized iron is selected for its reactivity, cost-effectiveness, adsorbing capacity eco-friendliness for contaminant removal from water. These are reported to be very helpful in removing contamination because of their area, size, and dispersion (Justin et al., 2017; Gupta et al., 2022). Kanel et al. (2006) conducted a comprehensive investigation on the application of nZVI across a broad spectrum of pH levels for the purpose of remediating As(V) contamination (Kanel et al., 2006). Another report demonstrated that nZVI exhibits notable reactivity, substantial surface modification, biocompatibility, and favorable magnetic properties (Xu et al., 2012).
Generally, nZVI exhibits the outer layer (Fe oxides) and inner layer Fe [0] in its structure. The inner layer (Fe [0]) reacts with water and oxidizes to form iron oxides, and finally forms different corrosion products like goethite, aragonite, and lepidocrocite (i.e., α-, β- and γ-FeOOH) (Mu et al., 2017). Liu et al. (2013) studied that all these corrosion products show excellent adsorption ability towards various pollutants. Wen et al. (2014) used a co-precipitation process and reported the phosphate adsorption capacity of 245.65 mg/g onto nZVI. Figure 7 shows the oxidation and reduction of various metallic compounds on the surface of nZVI, while Figure 8 shows the various forms of nZVI for environmental applications.
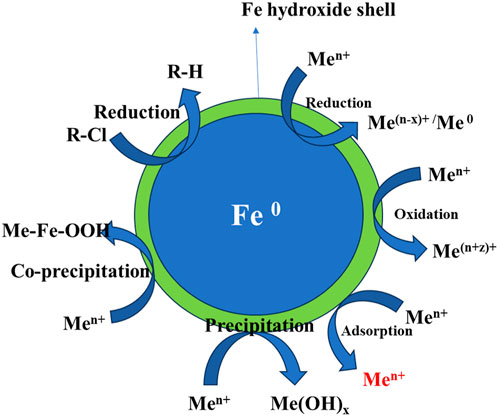
FIGURE 7. Core-shell structure of nZVI depicting various mechanisms for the removal of metals and chlorinated compounds reproduced from O’Carroll et al. (2013).
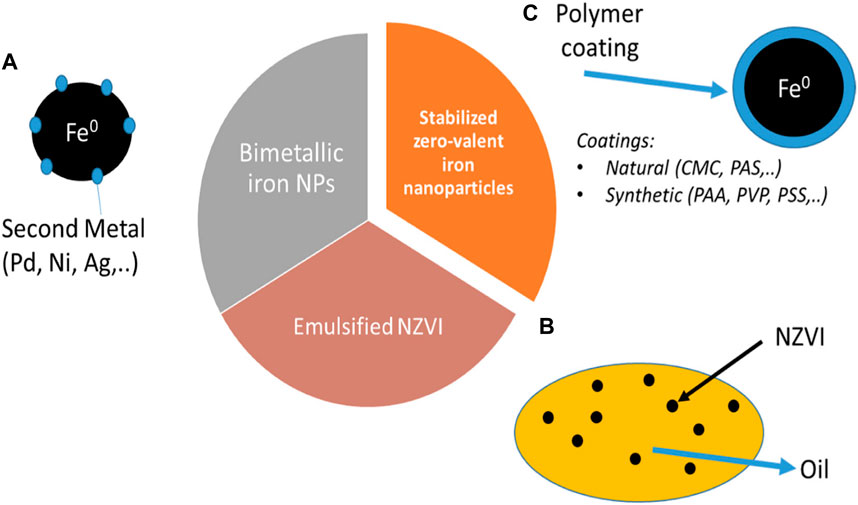
FIGURE 8. Main nZVI groups used for environmental applications: (A) Bimetallic iron nanoparticles, (B) emulsified nZVI, and (C) stabilized nZVI adapted from Galdames et al. (2020).
5.2.2 Nano-sized metal oxide
In recent years, nano-sized magnetic adsorbents have emerged as a significant field in nanoscience (Chen et al., 2022). Researchers studied nano-sized metal oxides towards various metallic contaminants like arsenic, cadmium, uranium, chromium, and phosphate toxins, and organics. Heavy metals, dichlorophenol, and MB were removed from water using a variety of nano-sized metal oxides that were all effective in their own ways (Chavali and Nikolova, 2019; Zhou et al., 2019; Gakis et al., 2023). Table 6 shows the applications of metal oxide NPs for removing wastewater pollutants.
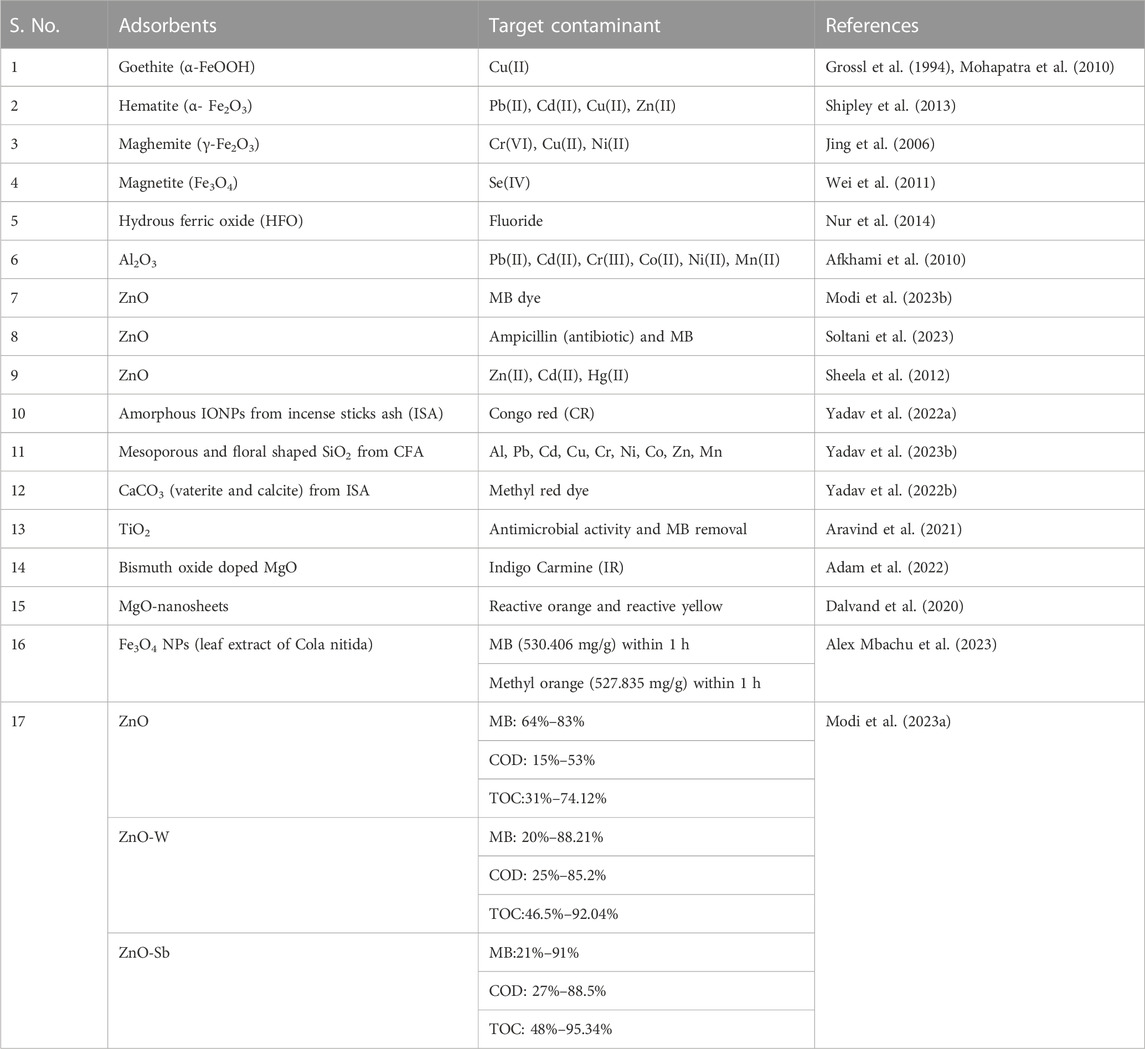
TABLE 6. Application of metal oxides-based nanoparticles for the remediation of wastewater pollutants.
Fagan et al. (2016) studied that endocrine-disrupting compounds, cyanotoxins, and antibiotics get removed by nano-sized metal oxide TiO2. Nano titanium oxide and copper oxide are utilized for electrocatalytic oxidation of organic compounds and chemical oxygen demand (COD) removal studied by Chang et al. (2009). In water purification, their pollutant removal utility was studied by various researchers, for example, pesticides, dyes, polymers, phenolic compounds, aldrin, polychlorinated biphenyls, etc. (Arabatzis et al., 2002; Cozzoli et al., 2004; Ahmed et al., 2011; Tolcha et al., 2020).
5.2.3 Noble metal nanoparticles
Certain transition metals (Au, Ag, Pt, and Pd) act as noble metals. The significant change in ionization energy and oxidation potential at the nanoscale range make them useful in many novel reactions. Organic contaminants are easily identified by gold and silver nanoparticles (AgNPs) because of their unique optical properties (Alberti et al., 2021; Nadaf et al., 2022). Noble metal NPs were synthesized by the reduction method through controlled nanocrystal nucleation with a stabilizing agent. The utilization of polymers and surfactants for enhancing stability was also demonstrated (Geng et al., 2022).
In the presence of pesticides, the gold nanoparticle surface will change with indoxyl groups at the ppt level. Contaminants are efficiently eliminated through the implementation of sensing, monitoring, and photocatalysis techniques facilitated by bimetallic nanoparticle-based electrodes (Behera et al., 2020; Rajeev et al., 2021; Białas et al., 2022). The role of the anti-bacterial activity and their sterilization effect (to sterilize surgical masks and textile fibers) was also studied (Xiu et al., 2011). Various pollutants like pesticides (Chaudhari et al., 2023), dyes, and halogenated compounds could be photo-catalytically degraded by noble metals (Quan et al., 2015).
5.3 Nanocomposites in water treatment
In the field of NMs, various nanocomposites were used as hosts and infused NPs and showed their significance in several reactions. Besides it, nanocomposites also reduce the environmental discharge of NPs (Hnamte and Pulikkal, 2022). These compact materials are used in the nanoscopic and mesoscopic scales, and their different varieties are discussed below.
5.3.1 Nanocomposites of organic supports
The unique characteristics of polymers, including porous structures, exceptional mechanical strength, and the presence of functional groups, make them highly suitable for use as supports in polymer-based nanocomposites (PNCs) for wastewater treatment. To eliminate heavy metal ions, PNCs (grafted magnetic nanoparticles) were prepared by grafting polymerization techniques (Uwamungu et al., 2022). Several research has been done on the fabrication of PNCs in which polymers and precursors of NPs are directly joined with NPs in direct compounding. They are synthesized by in-situ precipitation and nucleation methods. The potential applications and removal of contaminants of various nanocomposites are summarized in Table 7.
5.3.2 Nanocomposites of inorganic supports
For nanocomposites CNTs, naturally occurring minerals (zeolite, clay) and activated carbon are used as inorganic supports (Veeman et al., 2021). These adsorbents are extensively used in wastewater treatment facilities (Table 8).
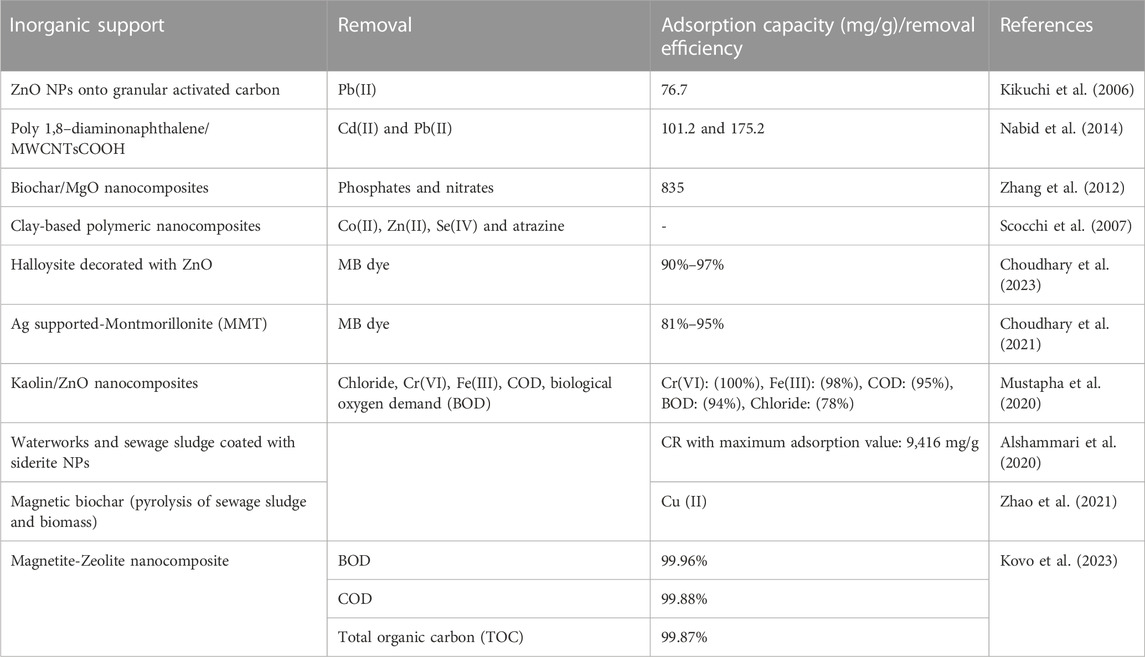
TABLE 8. Application of different inorganic support used for the removal of pollutants from wastewater.
5.3.3 Nanocomposite membrane for wastewater treatment
The unique properties of membranes such as long life, low cost, and high mechanical, chemical, and thermal stability, were used for water decontamination. Their low cost and less energy consumption make them useful at the industrial level (Shehata et al., 2023). They occur as conventional nanocomposite membranes, thin-film nanocomposites, and surface-coated nanocomposite membranes. The conventional nanocomposite membrane was prepared by the phase inversion method. A team led by Liu et al. (2015) studied the use of thin-film nanocomposite, in the RO/NF membrane through the phase inversion as well as the interfacial polymerization method. In surface-coated nanocomposites, NMs are used on the membrane surface by self-assembly, chemical grafting, in-situ deposition, and adsorption methods (Zhang et al., 2011b; Chaturvedi et al., 2022). Table 9 shows the recent development of inorganic and organic nanomembranes.
6 Conclusion
Nanotechnology and nanoparticles have played an important role in environmental cleanup and wastewater treatment in the 21st century. Due to its remarkable features, it has gained huge attention for the remediation of various organic pollutants like dyes, pesticides, heavy metals, pathogenic microorganisms, etc. The increase in the popularity of nanoparticles for remediation is due to their, surface-based phenomenon, high efficiency, and easy surface functionalization. To, date carbon NMs, metal, metal oxide nanoparticles, and nanocomposites have been used widely for wastewater treatment. The magnetic nanoparticles and photocatalytic nanoparticles are of huge importance as magnetic nanoparticles could be easily recovered while the photocatalytic materials could completely mineralize the toxic pollutants. Recovery after the application prevents the loss of the nanoparticles making the process highly effective. Indeed nanoparticles have a huge potential for the remediation of both organic and inorganic pollutants from wastewater.
Author contributions
MC, HS, VS, and NA: Original draft, review editing, methodology, software SK, DS, VY, and AP: Supervision, review editing, project administration, investigation, funding acquisition, resources. All authors contributed to the article and approved the submitted version.
Acknowledgments
All authors are thankful to the Department of Chemistry, Mody University of Science and Technology, and Department of Chemistry, ISR, IPS Academy Indore for providing basic facilities.
Conflict of interest
The authors declare that the research was conducted in the absence of any commercial or financial relationships that could be construed as a potential conflict of interest.
Publisher’s note
All claims expressed in this article are solely those of the authors and do not necessarily represent those of their affiliated organizations, or those of the publisher, the editors and the reviewers. Any product that may be evaluated in this article, or claim that may be made by its manufacturer, is not guaranteed or endorsed by the publisher.
References
Abbasi, M., Sabzehmeidani, M. M., Ghaedi, M., Jannesar, R., and Shokrollahi, A. (2021). Facile fabrication of leaf coral-like structured Cu-Al LDH/PVDF composite adsorptive membrane with enhanced adsorption performance. Mater. Sci. Eng. B 267, 115086. doi:10.1016/j.mseb.2021.115086
Abdelfatah, A. M., El-Maghrabi, N., Mahmoud, A. E. D., and Fawzy, M. (2022). Synergetic effect of green synthesized reduced graphene oxide and nano-zero valent iron composite for the removal of doxycycline antibiotic from water. Sci. Rep. 12, 19372. doi:10.1038/s41598-022-23684-x
Abdul Rahim, M. S., Ismail, I., and Aqida, S. N. (2017). Effects of nano copper additive on thermal conductivity of magnetorheological fluid at different environment temperature. Mater. Sci. Forum 890, 108–111. doi:10.4028/www.scientific.net/MSF.890.108
Abdulkareem, A. S., Hamzat, W. A., Tijani, J. O., Egbosiuba, T. C., Mustapha, S., Abubakre, O. K., et al. (2023). Isotherm, kinetics, thermodynamics and mechanism of metal ions adsorption from electroplating wastewater using treated and functionalized carbon nanotubes. J. Environ. Chem. Eng. 11, 109180. doi:10.1016/j.jece.2022.109180
Abdullah, N., Yusof, N., Abu Shah, M. H., Wan Ikhsan, S. N., Ng, Z.-C., Maji, S., et al. (2019). Hydrous ferric oxide nanoparticles hosted porous polyethersulfone adsorptive membrane: Chromium (VI) adsorptive studies and its applicability for water/wastewater treatment. Environ. Sci. Pollut. Res. 26, 20386–20399. doi:10.1007/s11356-019-05208-9
Adam, F. A., Ghoniem, M. G., Diawara, M., Rahali, S., Abdulkhair, B. Y., Elamin, M. R., et al. (2022). Enhanced adsorptive removal of indigo carmine dye by bismuth oxide doped MgO based adsorbents from aqueous solution: Equilibrium, kinetic and computational studies. RSC Adv. 12, 24786–24803. doi:10.1039/d2ra02636h
Adel, M., Ahmed, M. A., and Mohamed, A. A. (2021). A facile and rapid removal of cationic dyes using hierarchically porous reduced graphene oxide decorated with manganese ferrite. FlatChem 26, 100233. doi:10.1016/j.flatc.2021.100233
Adel, M., Ahmed, M. A., and Mohamed, A. A. (2020). Effective removal of cationic dyes from aqueous solutions using reduced graphene oxide functionalized with manganese ferrite nanoparticles. Compos. Commun. 22, 100450. doi:10.1016/j.coco.2020.100450
Afkhami, A., Saber-Tehrani, M., and Bagheri, H. (2010). Simultaneous removal of heavy-metal ions in wastewater samples using nano-alumina modified with 2,4-dinitrophenylhydrazine. J. Hazard Mater 181, 836–844. doi:10.1016/j.jhazmat.2010.05.089
Afolalu, S. A., Ikumapayi, O. M., Ogedengbe, T. S., Kazeem, R. A., and Ogundipe, A. T. (2022). Waste pollution, wastewater and effluent treatment methods – an overview. Mater Today Proc. 62, 3282–3288. doi:10.1016/j.matpr.2022.04.231
Ahmed, M., Mavukkandy, M. O., Giwa, A., Elektorowicz, M., Katsou, E., Khelifi, O., et al. (2022). Recent developments in hazardous pollutants removal from wastewater and water reuse within a circular economy. NPJ Clean. Water 5, 12. doi:10.1038/s41545-022-00154-5
Ahmed, S., Rasul, M. G., Brown, R., and Hashib, M. A. (2011). Influence of parameters on the heterogeneous photocatalytic degradation of pesticides and phenolic contaminants in wastewater: A short review. J. Environ. Manage 92, 311–330. doi:10.1016/j.jenvman.2010.08.028
Ai, L., and Jiang, J. (2012). Removal of methylene blue from aqueous solution with self-assembled cylindrical graphene–carbon nanotube hybrid. Chem. Eng. J. 192, 156–163. doi:10.1016/j.cej.2012.03.056
Ajiboye, T. O., Oladoye, P. O., Olanrewaju, C. A., and Akinsola, G. O. (2022). Organophosphorus pesticides: Impacts, detection and removal strategies. Environ. Nanotechnol. Monit. Manag. 17, 100655. doi:10.1016/j.enmm.2022.100655
Al-Asheh, S., and Aidan, A. (2020). “A comprehensive method of ion exchange resins regeneration and its optimization for water treatment,” in Promising techniques for wastewater treatment and water quality assessment. Editors I. A. Moujdin, and J. K. Summers (Rijeka: IntechOpen). doi:10.5772/intechopen.93429
Al-Musawi, T. J., Alghamdi, M. I., Alhachami, F. R., Zaidan, H., Mengelizadeh, N., Asghar, A., et al. (2023). The application of a new recyclable photocatalyst γ-Fe2O3@SiO2@ZIF8-Ag in the photocatalytic degradation of amoxicillin in aqueous solutions. Environ. Monit. Assess. 195, 372. doi:10.1007/s10661-023-10974-8
Alam, J., Yadav, V. K., Yadav, K. K., Cabral-Pinto, M. M. S., Tavker, N., Choudhary, N., et al. (2021). Recent advances in methods for the recovery of carbon nanominerals and polyaromatic hydrocarbons from coal fly ash and their emerging applications. Cryst. (Basel) 11, 88–24. doi:10.3390/cryst11020088
Alberti, G., Zanoni, C., Magnaghi, L. R., and Biesuz, R. (2021). Gold and silver nanoparticle-based colorimetric sensors: New trends and applications. Chemosensors 9, 305. doi:10.3390/chemosensors9110305
Alex Mbachu, C., Kamoru Babayemi, A., Chinedu Egbosiuba, T., Ifeanyichukwu Ike, J., Jacinta Ani, I., and Mustapha, S. (2023). Green synthesis of iron oxide nanoparticles by Taguchi design of experiment method for effective adsorption of methylene blue and methyl orange from textile wastewater. Results Eng. 19, 101198. doi:10.1016/j.rineng.2023.101198
Ali, S., Wali, A. F., Yatoo, A. M., Majid, S., Rasool, S., Khan, R., et al. (2020). “Effect of pesticides on fish fauna: Threats, challenges, and possible remedies,” in Bioremediation and biotechnology: Sustainable approaches to pollution degradation. Editors K. R. Hakeem, R. A. Bhat, and H. Qadri (Cham: Springer International Publishing), 27–54. doi:10.1007/978-3-030-35691-0_2
Aliyu, S., Ambali, A. S., Oladejo, T. J., Mustapha, S., Egbosiuba, T. C., and Bada, S. O. (2023). Development of Ag-doped on multi-walled carbon nanotubes for the treatment of fish pond effluent. Reg. Stud. Mar. Sci. 58, 102797. doi:10.1016/j.rsma.2022.102797
Almuallim, B., Harun, W. S. W., Al Rikabi, I. J., and Mohammed, H. A. (2022). Thermally conductive polymer nanocomposites for filament-based additive manufacturing. J. Mater Sci. 57, 3993–4019. doi:10.1007/s10853-021-06820-2
Alshammari, M., Al Juboury, M. F., Naji, L. A., Faisal, A. A. H., Zhu, H., Al-Ansari, N., et al. (2020). Synthesis of a novel composite sorbent coated with siderite nanoparticles and its application for remediation of water contaminated with Congo red dye. Int. J. Environ. Res. 14, 177–191. doi:10.1007/s41742-020-00245-6
Altug, H., Oh, S.-H., Maier, S. A., and Homola, J. (2022). Advances and applications of nanophotonic biosensors. Nat. Nanotechnol. 17, 5–16. doi:10.1038/s41565-021-01045-5
Amari, A., Yadav, V. K., Pathan, S. K., Singh, B., Osman, H., Choudhary, N., et al. (2023). Remediation of methyl red dye from aqueous solutions by using biosorbents developed from floral waste. Adsorpt. Sci. Technol. 2023, 1–17. doi:10.1155/2023/1532660
Amin, M. T., Alazba, A. A., and Manzoor, U. (2014). A review of removal of pollutants from water/wastewater using different types of nanomaterials. Adv. Mater. Sci. Eng. 2014, 1–24. doi:10.1155/2014/825910
Andronic, L., and Enesca, A. (2020). Black TiO2 synthesis by chemical reduction methods for photocatalysis applications. Front. Chem. 8, 565489. doi:10.3389/fchem.2020.565489
Andronic, L., Ghica, D., Stefan, M., Mihalcea, C. G., Vlaicu, A. M., and Karazhanov, S. (2022). Visible-Light-Active black TiO2 nanoparticles with efficient photocatalytic performance for degradation of pharmaceuticals. Nanomaterials 12, 2563. doi:10.3390/nano12152563
Aniculaesei, C., Pathak, V. B., Oh, K. H., Singh, P. K., Lee, B. R., Hojbota, C. I., et al. (2019). Proof-of-Principle experiment for nanoparticle-assisted laser wakefield electron acceleration. Phys. Rev. Appl. 12, 044041. doi:10.1103/PhysRevApplied.12.044041
Anjum, S., Ishaque, S., Fatima, H., Farooq, W., Hano, C., Abbasi, B. H., et al. (2021). Emerging applications of nanotechnology in healthcare systems: Grand challenges and perspectives. Pharmaceuticals 14, 707. doi:10.3390/ph14080707
Arabatzis, I. M., Antonaraki, S., Stergiopoulos, T., Hiskia, A., Papaconstantinou, E., Bernard, M. C., et al. (2002). Preparation, characterization and photocatalytic activity of nanocrystalline thin film TiO2 catalysts towards 3,5-dichlorophenol degradation. J. Photochem Photobiol. A Chem. 149, 237–245. doi:10.1016/S1010-6030(01)00645-1
Aragaw, T. A., and Ayalew, A. A. (2023). “Chapter 10 - application of metal-based nanoparticles for metal removal for treatments of wastewater -- a review,” in Emerging techniques for treatment of toxic metals from wastewater. Editors A. Ahmad, R. Kumar, and M. Jawaid (Netherlands: Elsevier), 183–231. doi:10.1016/B978-0-12-822880-7.00001-7
Aragaw, T. A., and Bogale, F. M. (2021). Biomass-based adsorbents for removal of dyes from wastewater: A review. Front. Environ. Sci. 9, 764958. doi:10.3389/fenvs.2021.764958
Aravind, M., Amalanathan, M., and Mary, M. S. M. (2021). Synthesis of TiO2 nanoparticles by chemical and green synthesis methods and their multifaceted properties. SN Appl. Sci. 3, 409. doi:10.1007/s42452-021-04281-5
Arshad, F., Selvaraj, M., Zain, J., Banat, F., and Haija, M. A. (2019). Polyethylenimine modified graphene oxide hydrogel composite as an efficient adsorbent for heavy metal ions. Sep. Purif. Technol. 209, 870–880. doi:10.1016/j.seppur.2018.06.035
Asif, M. B., and Zhang, Z. (2021). Ceramic membrane technology for water and wastewater treatment: A critical review of performance, full-scale applications, membrane fouling and prospects. Chem. Eng. J. 418, 129481. doi:10.1016/j.cej.2021.129481
Ba-Abbad, M. M., Benamour, A., Ewis, D., Mohammad, A. W., and Mahmoudi, E. (2022). Synthesis of Fe3O4 nanoparticles with different shapes through a Co-precipitation method and their application. JOM 74, 3531–3539. doi:10.1007/s11837-022-05380-3
Bagur, H., Medidi, R. S., Somu, P., Choudhury, P. W. J., karua, C. S., Guttula, P. K., et al. (2022). Endophyte fungal isolate mediated biogenic synthesis and evaluation of biomedical applications of silver nanoparticles. Mater. Technol. 37, 167–178. doi:10.1080/10667857.2020.1819089
Baig, N., Kammakakam, I., and Falath, W. (2021). Nanomaterials: A review of synthesis methods, properties, recent progress, and challenges. Mater Adv. 2, 1821–1871. doi:10.1039/D0MA00807A
Baig, U., Rao, R. A. K., Khan, A. A., Sanagi, M. M., and Gondal, M. A. (2015). Removal of carcinogenic hexavalent chromium from aqueous solutions using newly synthesized and characterized polypyrrole–titanium(IV)phosphate nanocomposite. Chem. Eng. J. 280, 494–504. doi:10.1016/j.cej.2015.06.031
Balarak, D., Zafariyan, M., Igwegbe, C. A., Onyechi, K. K., and Ighalo, J. O. (2021). Adsorption of acid blue 92 dye from aqueous solutions by single-walled carbon nanotubes: Isothermal, kinetic, and thermodynamic studies. Environ. Process. 8, 869–888. doi:10.1007/s40710-021-00505-3
Bangari, R. S., Yadav, A., Bharadwaj, J., and Sinha, N. (2022). Boron nitride nanosheets incorporated polyvinylidene fluoride mixed matrix membranes for removal of methylene blue from aqueous stream. J. Environ. Chem. Eng. 10, 107052. doi:10.1016/j.jece.2021.107052
Bantz, C., Koshkina, O., Lang, T., Galla, H. J., Kirkpatrick, C. J., Stauber, R. H., et al. (2014). The surface properties of nanoparticles determine the agglomeration state and the size of the particles under physiological conditions. Beilstein J. Nanotechnol. 5, 1774–1786. doi:10.3762/bjnano.5.188
Barakat, M. A. (2011). New trends in removing heavy metals from industrial wastewater. Arabian J. Chem. 4, 361–377. doi:10.1016/j.arabjc.2010.07.019
Batool, F., Iqbal, M. S., Khan, S.-U.-D., Khan, J., Ahmed, B., and Qadir, M. I. (2021). Biologically synthesized iron nanoparticles (FeNPs) from Phoenix dactylifera have anti-bacterial activities. Sci. Rep. 11, 22132. doi:10.1038/s41598-021-01374-4
Behera, A., Mittu, B., Padhi, S., Patra, N., and Singh, J. (2020). “Chapter 25 - bimetallic nanoparticles: Green synthesis, applications, and future perspectives,” in Multifunctional hybrid nanomaterials for sustainable agri-food and ecosystems. Editor K. A. Abd-Elsalam (Netherlands: Elsevier), 639–682. doi:10.1016/B978-0-12-821354-4.00025-X
Benko, H. (2017). “ISO technical committee 229 nanotechnologies,” in Metrology and standardization of nanotechnology (Netherlands: Elsevier), 259–268. doi:10.1002/9783527800308.ch14
Bharadwaj, V., Singh, N., and Sahoo, S. K. (2022). “Chapter 13 - polymeric nanoparticles with potential applications in sensing and biosensing,” in Sensing and biosensing with optically active nanomaterials. Editor S. K. Sahoo (Netherlands: Elsevier), 401–426. doi:10.1016/B978-0-323-90244-1.00001-X
Bhatnagar, A., and Sillanpää, M. (2009). Applications of chitin- and chitosan-derivatives for the detoxification of water and wastewater — a short review. Adv. Colloid Interface Sci. 152, 26–38. doi:10.1016/j.cis.2009.09.003
Bhol, P., Yadav, S., Altaee, A., Saxena, M., Misra, P. K., and Samal, A. K. (2021). Graphene-based membranes for water and wastewater treatment: A review. ACS Appl. Nano Mater 4, 3274–3293. doi:10.1021/acsanm.0c03439
Białas, K., Moschou, D., Marken, F., and Estrela, P. (2022). Electrochemical sensors based on metal nanoparticles with biocatalytic activity. Microchim. Acta 189, 172. doi:10.1007/s00604-022-05252-2
Bijekar, S., Padariya, H. D., Yadav, V. K., Gacem, A., Hasan, M. A., Awwad, N. S., et al. (2022). The state of the art and emerging trends in the wastewater treatment in developing nations. Water (Basel) 14, 2537. doi:10.3390/w14162537
Biswas, B., Labille, J., and Prelot, B. (2020). Clays and modified clays in remediating environmental pollutants. Environ. Sci. Pollut. Res. 27, 38381–38383. doi:10.1007/s11356-020-09828-4
Blaney, L. M., Cinar, S., and SenGupta, A. K. (2007). Hybrid anion exchanger for trace phosphate removal from water and wastewater. Water Res. 41, 1603–1613. doi:10.1016/j.watres.2007.01.008
Boparai, H. K., Joseph, M., and O’Carroll, D. M. (2011). Kinetics and thermodynamics of cadmium ion removal by adsorption onto nano zerovalent iron particles. J. Hazard Mater 186, 458–465. doi:10.1016/j.jhazmat.2010.11.029
Borse, V., and Konwar, A. N. (2020). Synthesis and characterization of gold nanoparticles as a sensing tool for the lateral flow immunoassay development. Sensors Int. 1, 100051. doi:10.1016/j.sintl.2020.100051
Bousiakou, L. G., Dobson, P. J., Jurkin, T., Marić, I., Aldossary, O., and Ivanda, M. (2022). Optical, structural and semiconducting properties of Mn doped TiO2 nanoparticles for cosmetic applications. J. King Saud. Univ. Sci. 34, 101818. doi:10.1016/j.jksus.2021.101818
Briffa, J., Sinagra, E., and Blundell, R. (2020). Heavy metal pollution in the environment and their toxicological effects on humans. Heliyon 6, e04691. doi:10.1016/j.heliyon.2020.e04691
Burk, G. A., Herath, A., Crisler, G. B., Bridges, D., Patel, S., Pittman, C. U., et al. (2020). Cadmium and copper removal from aqueous solutions using chitosan-coated gasifier biochar. Front. Environ. Sci. 8, 541203. doi:10.3389/fenvs.2020.541203
Cai, J., Zhang, Y., Pan, B., Zhang, W., Lv, L., and Zhang, Q. (2016). Efficient defluoridation of water using reusable nanocrystalline layered double hydroxides impregnated polystyrene anion exchanger. Water Res. 102, 109–116. doi:10.1016/j.watres.2016.06.030
Cao, K., Jiang, Z., Zhao, J., Zhao, C., Gao, C., Pan, F., et al. (2014). Enhanced water permeation through sodium alginate membranes by incorporating graphene oxides. J. Memb. Sci. 469, 272–283. doi:10.1016/j.memsci.2014.06.053
Chang, J.-H., Yang, T.-J., and Tung, C.-H. (2009). Performance of nano- and nonnano-catalytic electrodes for decontaminating municipal wastewater. J. Hazard Mater 163, 152–157. doi:10.1016/j.jhazmat.2008.06.072
Chaturvedi, A. K., Pappu, A., and Gupta, M. K. (2022). Unraveling the role of agro waste-derived graphene quantum dots on dielectric and mechanical property of the fly ash based polymer nanocomposite. J. Alloys Compd. 903, 163953. doi:10.1016/j.jallcom.2022.163953
Chaudhari, Y. S., Kumar, P., Soni, S., Gacem, A., Kumar, V., Singh, S., et al. (2023). An inclusive outlook on the fate and persistence of pesticides in the environment and integrated eco-technologies for their degradation. Toxicol. Appl. Pharmacol. 466, 116449. doi:10.1016/j.taap.2023.116449
Chavali, M. S., and Nikolova, M. P. (2019). Metal oxide nanoparticles and their applications in nanotechnology. SN Appl. Sci. 1, 607. doi:10.1007/s42452-019-0592-3
Chen, D., Sawut, A., and Wang, T. (2022). Synthesis of new functionalized magnetic nano adsorbents and adsorption performance for Hg(II) ions. Heliyon 8, e10528. doi:10.1016/j.heliyon.2022.e10528
Chen, X., Hong, L., Xu, Y., and Ong, Z. W. (2012). Ceramic pore channels with inducted carbon nanotubes for removing oil from water. ACS Appl. Mater Interfaces 4, 1909–1918. doi:10.1021/am300207b
Chen, Y., Pan, B., Li, H., Zhang, W., Lv, L., and Wu, J. (2010). Selective removal of Cu(II) ions by using cation-exchange resin-supported polyethyleneimine (PEI) nanoclusters. Environ. Sci. Technol. 44, 3508–3513. doi:10.1021/es100341x
Chiew, C. S. C., Poh, P. E., Pasbakhsh, P., Tey, B. T., Yeoh, H. K., and Chan, E. S. (2014). Physicochemical characterization of halloysite/alginate bionanocomposite hydrogel. Appl. Clay Sci. 101, 444–454. doi:10.1016/j.clay.2014.09.007
Chopra, H., Bibi, S., Singh, I., Hasan, M. M., Khan, M. S., Yousafi, Q., et al. (2022). Green metallic nanoparticles: Biosynthesis to applications. Front. Bioeng. Biotechnol. 10, 874742. doi:10.3389/fbioe.2022.874742
Choudhary, N., Yadav, V. K., Ali, H., Ali, D., Almutairi, B. O., Cavalu, S., et al. (2023). Remediation of methylene blue dye from wastewater by using zinc oxide nanoparticles loaded on nanoclay. WaterSwitzerl. 15, 1427. doi:10.3390/w15071427
Choudhary, N., Yadav, V. K., Yadav, K. K., Almohana, A. I., Almojil, S. F., Gnanamoorthy, G., et al. (2021). Application of green synthesized MMT/Ag nanocomposite for removal of methylene blue from aqueous solution. Water (Basel) 13, 3206. doi:10.3390/w13223206
Coccia, G., Tomassetti, S., and Di Nicola, G. (2021). Thermal conductivity of nanofluids: A review of the existing correlations and a scaled semi-empirical equation. Renew. Sustain. Energy Rev. 151, 111573. doi:10.1016/j.rser.2021.111573
Contini, C., Hindley, J. W., Macdonald, T. J., Barritt, J. D., Ces, O., and Quirke, N. (2020). Size dependency of gold nanoparticles interacting with model membranes. Commun. Chem. 3, 130. doi:10.1038/s42004-020-00377-y
Cozzoli, P. D., Comparelli, R., Fanizza, E., Curri, M. L., Agostiano, A., and Laub, D. (2004). Photocatalytic synthesis of silver nanoparticles stabilized by TiO2 nanorods: A semiconductor/metal nanocomposite in homogeneous nonpolar solution. J. Am. Chem. Soc. 126, 3868–3879. doi:10.1021/ja0395846
Crane, R. A., Dickinson, M., Popescu, I. C., and Scott, T. B. (2011). Magnetite and zero-valent iron nanoparticles for the remediation of uranium contaminated environmental water. Water Res. 45, 2931–2942. doi:10.1016/j.watres.2011.03.012
Cumbal, L., and SenGupta, A. K. (2005). Arsenic removal using polymer-supported hydrated iron(III) oxide nanoparticles: Role of donnan membrane effect. Environ. Sci. Technol. 39, 6508–6515. doi:10.1021/es050175e
Curvino, E. J., Chen, J. L., Permar, S. R., Fouda, G. G., and Collier, J. H. (2021). Advances in nanomaterial vaccine strategies to address infectious diseases impacting global health. Nat. Nanotechnol. 16, 1–14. doi:10.1038/s41565-020-0739-9
Czaplicka, N., Grzegórska, A., Wajs, J., Sobczak, J., and Rogala, A. (2021). Promising nanoparticle-based heat transfer fluids—Environmental and techno-economic analysis compared to conventional fluids. Int. J. Mol. Sci. 22, 9201. doi:10.3390/ijms22179201
Dalvand, R., Kianpour, E., Tahzibi, H., and Azizian, S. (2020). MgO nano-sheets for adsorption of anionic dyes from aqueous solution: Equilibrium and kinetics studies. Surfaces Interfaces 21, 100722. doi:10.1016/j.surfin.2020.100722
D’Ambrosio, C. N., Inchaussandague, M. E., and Skigin, D. C. (2022). Color properties of silver nanoparticle composites. Plasmonics 17, 31–42. doi:10.1007/s11468-021-01493-8
Das, S., Mukherjee, A., Sengupta, G., and Singh, V. K. (2020). “Chapter 18 - overview of nanomaterials synthesis methods, characterization techniques and effect on seed germination,” in Nano-materials as photocatalysts for degradation of environmental pollutants. Editors P. Singh, A. Borthakur, P. K. Mishra, and D. Tiwary (Netherlands: Elsevier), 371–401. doi:10.1016/B978-0-12-818598-8.00018-3
Dekker, F., Kool, L., Bunschoten, A., Velders, A. H., and Saggiomo, V. (2021). Syntheses of gold and silver dichroic nanoparticles; looking at the Lycurgus cup colors. Chem. Teach. Int. 3, 11. doi:10.1515/cti-2019-0011
Del Prado-Audelo, M. L., García Kerdan, I., Escutia-Guadarrama, L., Reyna-González, J. M., Magaña, J. J., and Leyva-Gómez, G. (2021). Nanoremediation: Nanomaterials and nanotechnologies for environmental cleanup. Front. Environ. Sci. 9, 793765. doi:10.3389/fenvs.2021.793765
DeMarco, M. J., SenGupta, A. K., and Greenleaf, J. E. (2003). Arsenic removal using a polymeric/inorganic hybrid sorbent. Water Res. 37, 164–176. doi:10.1016/S0043-1354(02)00238-5
Egbosiuba, T. C., and Abdulkareem, A. S. (2021). Highly efficient as-synthesized and oxidized multi-walled carbon nanotubes for copper(II) and zinc(II) ion adsorption in a batch and fixed-bed process. J. Mater. Res. Technol. 15, 2848–2872. doi:10.1016/j.jmrt.2021.09.094
Egbosiuba, T. C., Abdulkareem, A. S., Kovo, A. S., Afolabi, E. A., Tijani, J. O., and Roos, W. D. (2020). Enhanced adsorption of As(V) and Mn(VII) from industrial wastewater using multi-walled carbon nanotubes and carboxylated multi-walled carbon nanotubes. Chemosphere 254, 126780. doi:10.1016/j.chemosphere.2020.126780
Egbosiuba, T. C., Abdulkareem, A. S., Tijani, J. O., Ani, J. I., Krikstolaityte, V., Srinivasan, M., et al. (2021). Taguchi optimization design of diameter-controlled synthesis of multi walled carbon nanotubes for the adsorption of Pb(II) and Ni(II) from chemical industry wastewater. Chemosphere 266, 128937. doi:10.1016/j.chemosphere.2020.128937
Egbosiuba, T. C., Egwunyenga, M. C., Tijani, J. O., Mustapha, S., Abdulkareem, A. S., Kovo, A. S., et al. (2022). Activated multi-walled carbon nanotubes decorated with zero valent nickel nanoparticles for arsenic, cadmium and lead adsorption from wastewater in a batch and continuous flow modes. J. Hazard Mater 423, 126993. doi:10.1016/j.jhazmat.2021.126993
Eren, E., Sarihan, A., Eren, B., Gumus, H., and Kocak, F. O. (2015). Preparation, characterization and performance enhancement of polysulfone ultrafiltration membrane using PBI as hydrophilic modifier. J. Memb. Sci. 475, 1–8. doi:10.1016/j.memsci.2014.10.010
Erusappan, E., Thiripuranthagan, S., Radhakrishnan, R., Durai, M., Kumaravel, S., Vembuli, T., et al. (2021). Fabrication of mesoporous TiO2/PVDF photocatalytic membranes for efficient photocatalytic degradation of synthetic dyes. J. Environ. Chem. Eng. 9, 105776. doi:10.1016/j.jece.2021.105776
Facciotti, M., Boffa, V., Magnacca, G., Jørgensen, L. B., Kristensen, P. K., Farsi, A., et al. (2014). Deposition of thin ultrafiltration membranes on commercial SiC microfiltration tubes. Ceram. Int. 40, 3277–3285. doi:10.1016/j.ceramint.2013.09.107
Fagan, R., McCormack, D. E., Dionysiou, D. D., and Pillai, S. C. (2016). A review of solar and visible light active TiO2 photocatalysis for treating bacteria, cyanotoxins and contaminants of emerging concern. Mater Sci. Semicond. Process 42, 2–14. doi:10.1016/j.mssp.2015.07.052
Fan, J., Guo, Y., Wang, J., and Fan, M. (2009). Rapid decolorization of azo dye methyl orange in aqueous solution by nanoscale zerovalent iron particles. J. Hazard Mater 166, 904–910. doi:10.1016/j.jhazmat.2008.11.091
Fan, J., Zhang, B., Zhu, B., Shen, W., Chen, Y., and Zeng, F. (2023). New insight into the mechanism for the removal of methylene blue by hydrotalcite-supported nanoscale zero-valent iron. WaterSwitzerl. 15, 183. doi:10.3390/w15010183
Fan, X., Su, Y., Zhao, X., Li, Y., Zhang, R., Zhao, J., et al. (2014). Fabrication of polyvinyl chloride ultrafiltration membranes with stable antifouling property by exploring the pore formation and surface modification capabilities of polyvinyl formal. J. Memb. Sci. 464, 100–109. doi:10.1016/j.memsci.2014.04.005
Fang, Z., Qiu, X., Chen, J., and Qiu, X. (2011). Debromination of polybrominated diphenyl ethers by Ni/Fe bimetallic nanoparticles: Influencing factors, kinetics, and mechanism. J. Hazard Mater 185, 958–969. doi:10.1016/j.jhazmat.2010.09.113
Feng, J., Lang, G., Li, T., Zhang, J., Li, T., and Jiang, Z. (2022). Enhanced removal performance of zero-valent iron towards heavy metal ions by assembling Fe-tannin coating. J. Environ. Manage 319, 115619. doi:10.1016/j.jenvman.2022.115619
Fritea, L., Banica, F., Costea, T. O., Moldovan, L., Dobjanschi, L., Muresan, M., et al. (2021). Metal nanoparticles and carbon-based nanomaterials for improved performances of electrochemical (Bio)sensors with biomedical applications. Materials 14, 6319. doi:10.3390/ma14216319
Gacem, A., Modi, S., Yadav, V. K., Islam, S., Patel, A., Dawane, V., et al. (2022). Recent advances in methods for synthesis of carbon nanotubes and carbon nanocomposite and their emerging applications: A descriptive review. J. Nanomater 2022, 1–16. doi:10.1155/2022/7238602
Gadore, V., and Ahmaruzzaman, Md. (2021). Fly ash–based nanocomposites: A potential material for effective photocatalytic degradation/elimination of emerging organic pollutants from aqueous stream. Environ. Sci. Pollut. Res. 28, 46910–46933. doi:10.1007/s11356-021-15251-0
Gakis, G. P., Aviziotis, I. G., and Charitidis, C. A. (2023). Metal and metal oxide nanoparticle toxicity: Moving towards a more holistic structure–activity approach. Environ. Sci. Nano 10, 761–780. doi:10.1039/D2EN00897A
Galdames, A., Ruiz-Rubio, L., Orueta, M., Sánchez-Arzalluz, M., and Vilas-Vilela, J. L. (2020). Zero-valent iron nanoparticles for soil and groundwater remediation. Int. J. Environ. Res. Public Health 17, 5817–5823. doi:10.3390/ijerph17165817
Gao, Y., Li, Y., Zhang, L., Huang, H., Hu, J., Shah, S. M., et al. (2012). Adsorption and removal of tetracycline antibiotics from aqueous solution by graphene oxide. J. Colloid Interface Sci. 368, 540–546. doi:10.1016/j.jcis.2011.11.015
Gaur, V. K., Sharma, P., Gaur, P., Varjani, S., Ngo, H. H., Guo, W., et al. (2021). Sustainable mitigation of heavy metals from effluents: Toxicity and fate with recent technological advancements. Bioengineered 12, 7297–7313. doi:10.1080/21655979.2021.1978616
Geng, C., Zhao, F., Niu, H., Zhang, J., Dong, H., Li, Z., et al. (2022). Enhancing the permeability, anti-biofouling performance and long-term stability of TFC nanofiltration membrane by imidazole-modified carboxylated graphene oxide/polyethersulfone substrate. J. Memb. Sci. 664, 121099. doi:10.1016/j.memsci.2022.121099
Gnanamoorthy, G., Ali, D., Yadav, V. K., Dhinagaran, G., Venkatachalam, K., and Narayanan, V. (2020). New construction of Fe3O4/rGO/ZnSnO3 nanocomposites enhanced photoelectro chemical properties. Opt. Mater (Amst) 109, 110353. doi:10.1016/j.optmat.2020.110353
Gnanamoorthy, G., Karthikeyan, V., Ali, D., Kumar, G., Jenifer, S. G., Yadav, V. K., et al. (2021). Realization of rGO/ZnCo2O4 nanocomposites enhanced for the antimicrobial, electrochemical and photocatalytic activities. Diam. Relat. Mater 120, 108677. doi:10.1016/j.diamond.2021.108677
Gnanamoorthy, G., Karthikeyan, V., Ali, D., Kumar, G., Yadav, V. K., and Narayanan, V. (2022). Global popularization of CuNiO2 and their rGO nanocomposite loveabled to the photocatalytic properties of methylene blue. Environ. Res. 204, 112338. doi:10.1016/j.envres.2021.112338
Gopi, S., Balakrishnan, P., Pius, A., and Thomas, S. (2017). Chitin nanowhisker (ChNW)-functionalized electrospun PVDF membrane for enhanced removal of Indigo carmine. Carbohydr. Polym. 165, 115–122. doi:10.1016/j.carbpol.2017.02.046
Grossl, P. R., Sparks, D. L., and Ainsworth, C. C. (1994). Rapid kinetics of Cu(II) adsorption/desorption on goethite. Environ. Sci. Technol. 28, 1422–1429. doi:10.1021/es00057a008
Gu, S., Qin, M., Zhang, H., Ma, J., and Qu, X. (2018). Preparation of Mo nanopowders through hydrogen reduction of a combustion synthesized foam-like MoO2 precursor. Int. J. Refract Met. Hard Mater 76, 90–98. doi:10.1016/j.ijrmhm.2018.05.015
Guillem-Navajas, A., Martín-Illán, J. Á., Salagre, E., Michel, E. G., Rodriguez-San-Miguel, D., and Zamora, F. (2022). Iron oxyhydroxide-covalent organic framework nanocomposite for efficient as(III) removal in water. ACS Appl. Mater Interfaces 14, 50163–50170. doi:10.1021/acsami.2c14744
Guo, X., and Chen, F. (2005). Removal of arsenic by bead cellulose loaded with iron oxyhydroxide from groundwater. Environ. Sci. Technol. 39, 6808–6818. doi:10.1021/es048080k
Gupta, N., Yadav, V. K., Yadav, K. K., Alwetaishi, M., Gnanamoorthy, G., Singh, B., et al. (2022). Recovery of iron nanominerals from sacred incense sticks ash waste collected from temples by wet and dry magnetic separation method. Environ. Technol. Innov. 25, 102150. doi:10.1016/j.eti.2021.102150
Gupta, S., and Gupta, K. (2020). “Bioaccumulation of pesticides and its impact on biological systems,” in Pesticides in crop production (Netherlands: Elsevier), 55–67. doi:10.1002/9781119432241.ch4
Haleem, A., Javaid, M., Singh, R. P., Rab, S., and Suman, R. (2023). Applications of nanotechnology in medical field: A brief review. Glob. Health J. 7, 70–77. doi:10.1016/j.glohj.2023.02.008
Hammami, I., Alabdallah, N. M., Jomaa, A. A., and Kamoun, M. (2021). Gold nanoparticles: Synthesis properties and applications. J. King Saud. Univ. Sci. 33, 101560. doi:10.1016/j.jksus.2021.101560
Han, Y., Xu, Z., and Gao, C. (2013). Ultrathin graphene nanofiltration membrane for water purification. Adv. Funct. Mater 23, 3693–3700. doi:10.1002/adfm.201202601
Handayani, W., Pratiwi, N. I., Yulkifli, R., Benti Etika, S., and Imawan, C. (2019). A silver nanoparticle-based colorimetric detection of Fe2+. J. Phys. Conf. Ser. 1317, 012093. doi:10.1088/1742-6596/1317/1/012093
Hansen, S. F., Hansen, O. F. H., and Nielsen, M. B. (2020). Advances and challenges towards consumerization of nanomaterials. Nat. Nanotechnol. 15, 964–965. doi:10.1038/s41565-020-00819-7
Hartono, T., Wang, S., Ma, Q., and Zhu, Z. (2009). Layer structured graphite oxide as a novel adsorbent for humic acid removal from aqueous solution. J. Colloid Interface Sci. 333, 114–119. doi:10.1016/j.jcis.2009.02.005
He, C., Liu, Z., Wu, J., Pan, X., Fang, Z., Li, J., et al. (2021). Future global urban water scarcity and potential solutions. Nat. Commun. 12, 4667. doi:10.1038/s41467-021-25026-3
He, X., Zhang, K., Wang, H., Zhang, Y., Xiao, G., Niu, H., et al. (2022). Tailored carbon-based aramid nanofiber nanocomposites with highly anisotropic thermal conductivity and superior mechanical properties for thermal management. Carbon N. Y. 199, 367–378. doi:10.1016/j.carbon.2022.07.078
He, Z., Mahmud, S., Yang, Y., Zhu, L., Zhao, Y., Zeng, Q., et al. (2020). Polyvinylidene fluoride membrane functionalized with zero valent iron for highly efficient degradation of organic contaminants. Sep. Purif. Technol. 250, 117266. doi:10.1016/j.seppur.2020.117266
Heidarinejad, Z., Dehghani, M. H., Heidari, M., Javedan, G., Ali, I., and Sillanpää, M. (2020). Methods for preparation and activation of activated carbon: A review. Environ. Chem. Lett. 18, 393–415. doi:10.1007/s10311-019-00955-0
Hnamte, M., and Pulikkal, A. K. (2022). Clay-polymer nanocomposites for water and wastewater treatment: A comprehensive review. Chemosphere 307, 135869. doi:10.1016/j.chemosphere.2022.135869
Hong, J., and He, Y. (2014). Polyvinylidene fluoride ultrafiltration membrane blended with nano-ZnO particle for photo-catalysis self-cleaning. Desalination 332, 67–75. doi:10.1016/j.desal.2013.10.026
Horikoshi, S., and Serpone, N. (2013). “Introduction to nanoparticles,” in Microwaves in nanoparticle synthesis (Netherlands: Elsevier), 1–24. doi:10.1002/9783527648122.ch1
Hosseinifard, S. M., Aroon, M. A., and Dahrazma, B. (2020). Application of PVDF/HDTMA-modified clinoptilolite nanocomposite membranes in removal of reactive dye from aqueous solution. Sep. Purif. Technol. 251, 117294. doi:10.1016/j.seppur.2020.117294
Hu, M., and Mi, B. (2013). Enabling graphene oxide nanosheets as water separation membranes. Environ. Sci. Technol. 47, 3715–3723. doi:10.1021/es400571g
Huang, Z.-H., Zhang, X., Wang, Y.-X., Sun, J.-Y., Zhang, H., Liu, W.-L., et al. (2020). Fe3O4/PVDF catalytic membrane treatment organic wastewater with simultaneously improved permeability, catalytic property and anti-fouling. Environ. Res. 187, 109617. doi:10.1016/j.envres.2020.109617
Huang, Z., Luo, N., Zhang, C., and Wang, F. (2022). Radical generation and fate control for photocatalytic biomass conversion. Nat. Rev. Chem. 6, 197–214. doi:10.1038/s41570-022-00359-9
Ibrahim, S. I., Ali, A. H., Hafidh, S. A., Chaichan, M. T., Kazem, H. A., Ali, J. M., et al. (2022). Stability and thermal conductivity of different nano-composite material prepared for thermal energy storage applications. S Afr. J. Chem. Eng. 39, 72–89. doi:10.1016/j.sajce.2021.11.010
Igwegbe, C. A., Ighalo, J. O., Onyechi, K. K., and Onukwuli, O. D. (2021). Adsorption of Congo red and malachite green using H3PO4 and NaCl-modified activated carbon from rubber (Hevea brasiliensis) seed shells. Sustain Water Resour. Manag. 7, 63. doi:10.1007/s40899-021-00544-6
Inamdar, A. K., Rajenimbalkar, R. S., Hulsure, N. R., Kadam, A. S., Shinde, B. H., Patole, S. P., et al. (2023). A review on environmental applications of metal oxide nanoparticles through waste water treatment. Mater Today Proc. 2023, 527. doi:10.1016/j.matpr.2023.05.527
Irannajad, M., and Kamran Haghighi, H. (2021). Removal of heavy metals from polluted solutions by zeolitic adsorbents: A review. Environ. Process. 8, 7–35. doi:10.1007/s40710-020-00476-x
Isawi, H. (2020). Using Zeolite/Polyvinyl alcohol/sodium alginate nanocomposite beads for removal of some heavy metals from wastewater. Arabian J. Chem. 13, 5691–5716. doi:10.1016/j.arabjc.2020.04.009
Islam, T., Repon, Md. R., Islam, T., Sarwar, Z., and Rahman, M. M. (2023). Impact of textile dyes on health and ecosystem: A review of structure, causes, and potential solutions. Environ. Sci. Pollut. Res. 30, 9207–9242. doi:10.1007/s11356-022-24398-3
Jagadeesh, D., Prashantha, K., and Shabadi, R. (2017). Star-shaped sucrose-capped CaO nanoparticles from Azadirachta indica: A novel green synthesis. Inorg. Nano-Metal Chem. 47, 708–712. doi:10.1080/15533174.2016.1212231
Jahankhah, S., Sabzehmeidani, M. M., Ghaedi, M., Dashtian, K., and Abbasi-Asl, H. (2021). Hydrophilic magnetic molecularly imprinted resin in PVDF membrane for efficient selective removal of dye. J. Environ. Manage 300, 113707. doi:10.1016/j.jenvman.2021.113707
Jaiswal, M., Chauhan, D., and Sankararamakrishnan, N. (2012). Copper chitosan nanocomposite: Synthesis, characterization, and application in removal of organophosphorous pesticide from agricultural runoff. Environ. Sci. Pollut. Res. 19, 2055–2062. doi:10.1007/s11356-011-0699-6
Jefremovas, E. M., Gandarias, L., Rodrigo, I., Marcano, L., Grüttner, C., García, J. Á., et al. (2021). Nanoflowers versus magnetosomes: Comparison between two promising candidates for magnetic hyperthermia therapy. IEEE Access 9, 99552–99561. doi:10.1109/ACCESS.2021.3096740
Jia, Y., Hou, X., Wang, Z., and Hu, X. (2021). Machine learning boosts the design and discovery of nanomaterials. ACS Sustain Chem. Eng. 9, 6130–6147. doi:10.1021/acssuschemeng.1c00483
Jiang, Z., Lv, L., Zhang, W., Du, Q., Pan, B., Yang, L., et al. (2011). Nitrate reduction using nanosized zero-valent iron supported by polystyrene resins: Role of surface functional groups. Water Res. 45, 2191–2198. doi:10.1016/j.watres.2011.01.005
Jin, R., and Higaki, T. (2021). Open questions on the transition between nanoscale and bulk properties of metals. Commun. Chem. 4, 28. doi:10.1038/s42004-021-00466-6
Jing, H., Guohua, C., and C, L. I. M. (2006). Selective removal of heavy metals from industrial wastewater using maghemite nanoparticle: Performance and mechanisms. J. Environ. Eng. 132, 709–715. doi:10.1061/(asce)0733-9372(2006)132:7(709)
Justin, C., Philip, S. A., and Samrot, A. V. (2017). Synthesis and characterization of superparamagnetic iron-oxide nanoparticles (SPIONs) and utilization of SPIONs in X-ray imaging. Appl. Nanosci. Switz. 7, 463–475. doi:10.1007/s13204-017-0583-x
Kabir, R., Saifullah, M. A. K., Ahmed, A. Z., Masum, S. M., and Molla, M. A. I. (2020). Synthesis of n-doped zno nanocomposites for sunlight photocatalytic degradation of textile dye pollutants. J. Compos. Sci. 4, 49. doi:10.3390/jcs4020049
Kanel, S. R., Grenèche, J.-M., and Choi, H. (2006). Arsenic(V) removal from groundwater using nano scale zero-valent iron as a colloidal reactive barrier material. Environ. Sci. Technol. 40, 2045–2050. doi:10.1021/es0520924
Katsoyiannis, I. A., and Zouboulis, A. I. (2002). Removal of arsenic from contaminated water sources by sorption onto iron-oxide-coated polymeric materials. Water Res. 36, 5141–5155. doi:10.1016/S0043-1354(02)00236-1
Kaur, J., Kaur, K., Pervaiz, N., and Mehta, S. K. (2021). Spherical MoO3 nanoparticles for photocatalytic removal of eriochrome black T. ACS Appl. Nano Mater 4, 12766–12778. doi:10.1021/acsanm.1c03433
Kaurav, M., Ruhi, S., Al-Goshae, H. A., Jeppu, A. K., Ramachandran, D., Sahu, R. K., et al. (2023). Dendrimer: An update on recent developments and future opportunities for the brain tumors diagnosis and treatment. Front. Pharmacol. 14, 1159131. doi:10.3389/fphar.2023.1159131
Kawada, S., Saeki, D., and Matsuyama, H. (2014). Development of ultrafiltration membrane by stacking of silver nanoparticles stabilized with oppositely charged polyelectrolytes. Colloids Surf. A Physicochem Eng. Asp. 451, 33–37. doi:10.1016/j.colsurfa.2014.03.043
Khan, I., Saeed, K., and Khan, I. (2019). Nanoparticles: Properties, applications and toxicities. Arabian J. Chem. 12, 908–931. doi:10.1016/j.arabjc.2017.05.011
Khan, M., Khan, A. A., Parveen, A., Min, K., Yadav, V. K., Khan, A. U., et al. (2023). Mitigating the growth of plant pathogenic bacterium, fungi, and nematode by using plant-mediated synthesis of copper oxide nanoparticles (CuO NPs). Green Chem. Lett. Rev. 16, 2177520. doi:10.1080/17518253.2023.2177520
Khan, M. M. (2021). “Chapter 1 - principles and mechanisms of photocatalysis,” in Photocatalytic systems by design. Editors M. Sakar, R. G. Balakrishna, and T.-O. Do (Netherlands: Elsevier), 1–22. doi:10.1016/B978-0-12-820532-7.00008-4
Kikuchi, Y., Qian, Q., Machida, M., and Tatsumoto, H. (2006). Effect of ZnO loading to activated carbon on Pb(II) adsorption from aqueous solution. Carbon N. Y. 44, 195–202. doi:10.1016/j.carbon.2005.07.040
König, K., Boffa, V., Buchbjerg, B., Farsi, A., Christensen, M. L., Magnacca, G., et al. (2014). One-step deposition of ultrafiltration SiC membranes on macroporous SiC supports. J. Memb. Sci. 472, 232–240. doi:10.1016/j.memsci.2014.08.058
Kovo, A. S., Alaya-Ibrahim, S., Abdulkareem, A. S., Adeniyi, O. D., Egbosiuba, T. C., Tijani, J. O., et al. (2023). Column adsorption of biological oxygen demand, chemical oxygen demand and total organic carbon from wastewater by magnetite nanoparticles-zeolite A composite. Heliyon 9, e13095. doi:10.1016/j.heliyon.2023.e13095
Kumanek, B., and Janas, D. (2019). Thermal conductivity of carbon nanotube networks: A review. J. Mater Sci. 54, 7397–7427. doi:10.1007/s10853-019-03368-0
Kumar, S., Anwer, R., Sehrawat, A., Yadav, M., and Sehrawat, N. (2021). Assessment of bacterial pathogens in drinking water: A serious safety concern. Curr. Pharmacol. Rep. 7, 206–212. doi:10.1007/s40495-021-00263-8
Kumar, S., Nair, R. R., Pillai, P. B., Gupta, S. N., Iyengar, M. A. R., and Sood, A. K. (2014). Graphene oxide–MnFe2O4 magnetic nanohybrids for efficient removal of lead and arsenic from water. ACS Appl. Mater Interfaces 6, 17426–17436. doi:10.1021/am504826q
Kumar Yadav, V., Singh, B., Gacem, A., Kumar Yadav, K., Gnanamoorthy, G., Alsufyani, T., et al. (2022). Development of novel microcomposite materials from coal fly ash and incense sticks ash waste and their application for remediation of malachite green dye from aqueous solutions. Water 14, 3871. doi:10.3390/w14233871
Levofloxacin, D., Hu, B., Yang, L., Al-Musawi, T. J., Qasim Almajidi, Y., Al-Essa, E. M., et al. (2022). Levofloxacin adsorption onto MWCNTs/CoFe2O4 nanocomposites: Mechanism, and modeling using non-linear kinetics and isotherm equations. Magnetochemistry 9, 9. doi:10.3390/magnetochemistry9010009
Li, S., Wang, W., Yan, W., and Zhang, W. (2014). Nanoscale zero-valent iron (nZVI) for the treatment of concentrated Cu(ii) wastewater: A field demonstration. Environ. Sci. Process Impacts 16, 524–533. doi:10.1039/C3EM00578J
Li, X., Elliott, D. W., and Zhang, W. (2006). Zero-valent iron nanoparticles for abatement of environmental pollutants: Materials and engineering aspects. Crit. Rev. Solid State Mater. Sci. 31, 111–122. doi:10.1080/10408430601057611
Li, X., Yang, W., Li, H., Wang, Y., Bubakir, M. M., Ding, Y., et al. (2015). Water filtration properties of novel composite membranes combining solution electrospinning and needleless melt electrospinning methods. J. Appl. Polym. Sci. 132. doi:10.1002/app.41601
Li, Y., Zhou, Y., Zhou, Y., Lei, J., and Pu, S. (2019). Cyclodextrin modified filter paper for removal of cationic dyes/Cu ions from aqueous solutions. Water Sci. Technol. 78, 2553–2563. doi:10.2166/wst.2019.009
Lin, B.-R., Chen, C.-H., Kunuku, S., Chen, T.-Y., Hsiao, T.-Y., Niu, H., et al. (2018). Fe doped magnetic nanodiamonds made by ion implantation as contrast agent for MRI. Sci. Rep. 8, 7058. doi:10.1038/s41598-018-25380-1
Liu, D., Yin, J., Tang, H., Wang, H., Liu, S., Huang, T., et al. (2021a). Fabrication of ZIF-67@PVDF ultrafiltration membrane with improved antifouling and separation performance for dye wastewater treatment via sulfate radical enhancement. Sep. Purif. Technol. 279, 119755. doi:10.1016/j.seppur.2021.119755
Liu, D., Zhang, A., Wang, R., Zhang, Q., and Cui, D. (2021b). A review on metal- and metal oxide-based nanozymes: Properties, mechanisms, and applications. Nanomicro Lett. 13, 154. doi:10.1007/s40820-021-00674-8
Liu, G., Jin, W., and Xu, N. (2015). Graphene-based membranes. Chem. Soc. Rev. 44, 5016–5030. doi:10.1039/C4CS00423J
Liu, L., Li, C., Bao, C., Jia, Q., Xiao, P., Liu, X., et al. (2012a). Preparation and characterization of chitosan/graphene oxide composites for the adsorption of Au(III) and Pd(II). Talanta 93, 350–357. doi:10.1016/j.talanta.2012.02.051
Liu, L., Liu, S., Zhang, Q., Li, C., Bao, C., Liu, X., et al. (2013). Adsorption of Au(III), Pd(II), and Pt(IV) from aqueous solution onto graphene oxide. J. Chem. Eng. Data 58, 209–216. doi:10.1021/je300551c
Liu, L., Liu, Z., Bai, H., and Sun, D. D. (2012b). Concurrent filtration and solar photocatalytic disinfection/degradation using high-performance Ag/TiO2 nanofiber membrane. Water Res. 46, 1101–1112. doi:10.1016/j.watres.2011.12.009
Liu, T., Wang, P., and Wang, Z.-L. (2022). A high-efficient and recyclable aged nanoscale zero-valent iron compound for V5+ removal from wastewater: Characterization, performance and mechanism. Chemosphere 302, 134833. doi:10.1016/j.chemosphere.2022.134833
Liu, W., Sun, W., Borthwick, A. G. L., Wang, T., Li, F., and Guan, Y. (2016). Simultaneous removal of Cr(VI) and 4-chlorophenol through photocatalysis by a novel anatase/titanate nanosheet composite: Synergetic promotion effect and autosynchronous doping. J. Hazard Mater 317, 385–393. doi:10.1016/j.jhazmat.2016.06.002
Lu, C., and Chiu, H. (2006). Adsorption of zinc(II) from water with purified carbon nanotubes. Chem. Eng. Sci. 61, 1138–1145. doi:10.1016/j.ces.2005.08.007
Lu, C., and Liu, C. (2006). Removal of nickel(II) from aqueous solution by carbon nanotubes. J. Chem. Technol. Biotechnol. 81, 1932–1940. doi:10.1002/jctb.1626
Lu, P., Wu, X., Guo, W., and Zeng, X. C. (2012). Strain-dependent electronic and magnetic properties of MoS2 monolayer, bilayer, nanoribbons and nanotubes. Phys. Chem. Chem. Phys. 14, 13035–13040. doi:10.1039/C2CP42181J
Luo, X., Wang, C., Wang, L., Deng, F., Luo, S., Tu, X., et al. (2013). Nanocomposites of graphene oxide-hydrated zirconium oxide for simultaneous removal of As(III) and As(V) from water. Chem. Eng. J. 220, 98–106. doi:10.1016/j.cej.2013.01.017
Ma, D., Yi, H., Lai, C., Liu, X., Huo, X., An, Z., et al. (2021a). Critical review of advanced oxidation processes in organic wastewater treatment. Chemosphere 275, 130104. doi:10.1016/j.chemosphere.2021.130104
Ma, D., Zhan, Y., Zhang, Y., Mao, C., Xie, X., and Lin, Y. (2021b). The biological applications of DNA nanomaterials: Current challenges and future directions. Signal Transduct. Target Ther. 6, 351. doi:10.1038/s41392-021-00727-9
Madadrang, C. J., Kim, H. Y., Gao, G., Wang, N., Zhu, J., Feng, H., et al. (2012). Adsorption behavior of EDTA-graphene oxide for Pb (II) removal. ACS Appl. Mater Interfaces 4, 1186–1193. doi:10.1021/am201645g
Mahmoud, K. A., Mansoor, B., Mansour, A., and Khraisheh, M. (2015). Functional graphene nanosheets: The next generation membranes for water desalination. Desalination 356, 208–225. doi:10.1016/j.desal.2014.10.022
Maliki, M., Ifijen, I. H., Ikhuoria, E. U., Jonathan, E. M., Onaiwu, G. E., Archibong, U. D., et al. (2022). Copper nanoparticles and their oxides: Optical, anticancer and antibacterial properties. Int. Nano Lett. 12, 379–398. doi:10.1007/s40089-022-00380-2
Malla, M. A., Gupta, S., Dubey, A., Kumar, A., and Yadav, S. (2021). “Chapter 7 - contamination of groundwater resources by pesticides,” in Contamination of water. Editors A. Ahamad, S. I. Siddiqui, and P. Singh (United States: Academic Press), 99–107. doi:10.1016/B978-0-12-824058-8.00023-2
Manickam, N. K., Kandasamy, S., Jayabharathi, J., Samraj, S., and Sangeetha Gandhi, S. (2021). “Chapter 3 - sustainable energy production using nanomaterials and nanotechnology,” in Nanomaterials. Editors R. P. Kumar, and B. Bharathiraja (United States: Academic Press), 57–62. doi:10.1016/B978-0-12-822401-4.00037-4
Manju, G. N., Anoop Krishnan, K., Vinod, V. P., and Anirudhan, T. S. (2002). An investigation into the sorption of heavy metals from wastewaters by polyacrylamide-grafted iron(III) oxide. J. Hazard Mater 91, 221–238. doi:10.1016/S0304-3894(01)00392-2
Mansur, A. A. P., Mansur, H. S., Ramanery, F. P., Oliveira, L. C., and Souza, P. P. (2014). Green colloidal ZnS quantum dots/chitosan nano-photocatalysts for advanced oxidation processes: Study of the photodegradation of organic dye pollutants. Appl. Catal. B 158–159, 269–279. doi:10.1016/j.apcatb.2014.04.026
Materón, E. M., Miyazaki, C. M., Carr, O., Joshi, N., Picciani, P. H. S., Dalmaschio, C. J., et al. (2021). Magnetic nanoparticles in biomedical applications: A review. Appl. Surf. Sci. Adv. 6, 100163. doi:10.1016/j.apsadv.2021.100163
Mehdizadeh, S., Sadjadi, S., Ahmadi, S. J., and Outokesh, M. (2014). Removal of heavy metals from aqueous solution using platinum nanopartcles/Zeolite-4A. J. Environ. Health Sci. Eng. 12, 7. doi:10.1186/2052-336X-12-7
Mishra, S., Sahu, A., Dungdung, M., Ahmed, S., and Baitharu, I. (2023). “Chapter 2 - pesticide pollution in freshwater and its impact on community health,” in Current developments in biotechnology and bioengineering. Editors J. Singh, A. Pandey, S. Singh, V. K. Garg, and P. Ramamurthy (Netherlands: Elsevier), 33–52. doi:10.1016/B978-0-323-91900-5.00005-9
Modi, S., Yadav, V. K., Amari, A., Alyami, A. Y., Gacem, A., Harharah, H. N., et al. (2023a). Photocatalytic degradation of methylene blue dye from wastewater by using doped zinc oxide nanoparticles. Water (Basel) 15, 2275. doi:10.3390/w15122275
Modi, S., Yadav, V. K., Amari, A., Osman, H., Igwegbe, C. A., and Fulekar, M. H. (2023b). Nanobioremediation: A bacterial consortium-zinc oxide nanoparticle-based approach for the removal of methylene blue dye from wastewater. Environ. Sci. Pollut. Res. 30, 72641–72651. doi:10.1007/s11356-023-27507-y
Modi, S., Yadav, V. K., Gacem, A., Ali, I. H., Dave, D., Khan, S. H., et al. (2022). Recent and emerging trends in remediation of methylene blue dye from wastewater by using zinc oxide nanoparticles. Water 14, 1749. doi:10.3390/w14111749
Mohapatra, M., Rout, K., Gupta, S. K., Singh, P., Anand, S., and Mishra, B. K. (2010). Facile synthesis of additive-assisted nano goethite powder and its application for fluoride remediation. J. Nanoparticle Res. 12, 681–686. doi:10.1007/s11051-009-9779-7
Mondal, N. K., and Chakraborty, S. (2020). Adsorption of Cr(VI) from aqueous solution on graphene oxide (GO) prepared from graphite: Equilibrium, kinetic and thermodynamic studies. Appl. Water Sci. 10, 61. doi:10.1007/s13201-020-1142-2
Mu, Y., Jia, F., Ai, Z., and Zhang, L. (2017). Iron oxide shell mediated environmental remediation properties of nano zero-valent iron. Environ. Sci. Nano 4, 27–45. doi:10.1039/C6EN00398B
Murthy, H. C. A., Desalegn, T., Kassa, M., Abebe, B., and Assefa, T. (2020). Synthesis of green copper nanoparticles using medicinal plant hagenia abyssinica (brace) JF. Gmel. Leaf extract: Antimicrobial properties. J. Nanomater 2020, 1–12. doi:10.1155/2020/3924081
Murukutti, M. K., and Jena, H. (2022). Synthesis of nano-crystalline zeolite-A and zeolite-X from Indian coal fly ash, its characterization and performance evaluation for the removal of Cs+ and Sr2+ from simulated nuclear waste. J. Hazard Mater 423, 127085. doi:10.1016/j.jhazmat.2021.127085
Mustapha, S., Tijani, J. O., Ndamitso, M. M., Abdulkareem, S. A., Shuaib, D. T., Mohammed, A. K., et al. (2020). The role of kaolin and kaolin/ZnO nanoadsorbents in adsorption studies for tannery wastewater treatment. Sci. Rep. 10, 13068. doi:10.1038/s41598-020-69808-z
Nabid, M. R., Sedghi, R., Behbahani, M., Arvan, B., Heravi, M. M., and Oskooie, H. A. (2014). Application of Poly 1,8-diaminonaphthalene/multiwalled carbon nanotubes-COOH hybrid material as an efficient sorbent for trace determination of cadmium and lead ions in water samples. J. Mol. Recognit. 27, 421–428. doi:10.1002/jmr.2361
Nadaf, S. J., Jadhav, N. R., Naikwadi, H. S., Savekar, P. L., Sapkal, I. D., Kambli, M. M., et al. (2022). Green synthesis of gold and silver nanoparticles: Updates on research, patents, and future prospects. OpenNano 8, 100076. doi:10.1016/j.onano.2022.100076
Naseem, T., and Durrani, T. (2021). The role of some important metal oxide nanoparticles for wastewater and antibacterial applications: A review. Environ. Chem. Ecotoxicol. 3, 59–75. doi:10.1016/j.enceco.2020.12.001
Nasreen, S. A. A. N., Sundarrajan, S., Syed Nizar, S. A., Balamurugan, R., and Ramakrishna, S. (2014). In situ polymerization of PVDF-HEMA polymers: Electrospun membranes with improved flux and antifouling properties for water filtration. Polym. J. 46, 167–174. doi:10.1038/pj.2013.79
Nazari, L., Xu, C., Charles), , and Ray, M. B. (2021). “Nitrogen and phosphorous recovery from municipal wastewater and sludge,” in Advanced and emerging technologies for resource recovery from wastes. Editors L. Nazari, C. Charles Xu, and M. B. Ray (Singapore: Springer Singapore), 97–125. doi:10.1007/978-981-15-9267-6_4
Nile, S. H., Baskar, V., Selvaraj, D., Nile, A., Xiao, J., and Kai, G. (2020). Nanotechnologies in food science: Applications, recent trends, and future perspectives. Nanomicro Lett. 12, 45. doi:10.1007/s40820-020-0383-9
Nur, T., Loganathan, P., Nguyen, T. C., Vigneswaran, S., Singh, G., and Kandasamy, J. (2014). Batch and column adsorption and desorption of fluoride using hydrous ferric oxide: Solution chemistry and modeling. Chem. Eng. J. 247, 93–102. doi:10.1016/j.cej.2014.03.009
O’Carroll, D., Sleep, B., Krol, M., Boparai, H., and Kocur, C. (2013). Nanoscale zero valent iron and bimetallic particles for contaminated site remediation. Adv. Water Resour. 51, 104–122. doi:10.1016/j.advwatres.2012.02.005
Pan, B., Ren, R., Liang, B., Li, L., Li, H., and Cao, B. (2014b). Synthesis of pH-responsive polyethylene terephthalate track-etched membranes by grafting hydroxyethyl-methacrylate using atom-transfer radical polymerization method. J. Appl. Polym. Sci. 131. doi:10.1002/app.40912
Pan, B., Wan, S., Zhang, S., Guo, Q., Xu, Z., Lv, L., et al. (2014a). Recyclable polymer-based nano-hydrous manganese dioxide for highly efficient Tl(I) removal from water. Sci. China Chem. 57, 763–771. doi:10.1007/s11426-013-4992-8
Parveen, F., Sannakki, B., Mandke, M. V., and Pathan, H. M. (2016). Copper nanoparticles: Synthesis methods and its light harvesting performance. Sol. Energy Mater. Sol. Cells 144, 371–382. doi:10.1016/j.solmat.2015.08.033
Pezeshk, N., Rana, D., Narbaitz, R. M., and Matsuura, T. (2012). Novel modified PVDF ultrafiltration flat-sheet membranes. J. Memb. Sci. 389, 280–286. doi:10.1016/j.memsci.2011.10.039
Phillips, J. D. (2021). Energy harvesting in nanosystems: Powering the next generation of the internet of things. Front. Nanotechnol. 3. doi:10.3389/fnano.2021.633931
Pokrajac, L., Abbas, A., Chrzanowski, W., Dias, G. M., Eggleton, B. J., Maguire, S., et al. (2021). Nanotechnology for a sustainable future: Addressing global challenges with the international Network4Sustainable nanotechnology. ACS Nano 15, 18608–18623. doi:10.1021/acsnano.1c10919
Pomerantseva, E., Bonaccorso, F., Feng, X., Cui, Y., and Gogotsi, Y. (2019). Energy storage: The future enabled by nanomaterials. Science 366, eaan8285. doi:10.1126/science.aan8285
Pourabadeh, A., Baharinikoo, L., Shojaei, S., Mehdizadeh, B., Davoodabadi Farahani, M., and Shojaei, S. (2020). Experimental design and modelling of removal of dyes using nano-zero-valent iron: A simultaneous model. Int. J. Environ. Anal. Chem. 100, 1707–1719. doi:10.1080/03067319.2019.1657855
Priyadarshini, M., Das, I., Ghangrekar, M. M., and Blaney, L. (2022). Advanced oxidation processes: Performance, advantages, and scale-up of emerging technologies. J. Environ. Manage 316, 115295. doi:10.1016/j.jenvman.2022.115295
Puri, N., Gupta, A., and Mishra, A. (2021). Recent advances on nano-adsorbents and nanomembranes for the remediation of water. J. Clean. Prod. 322, 129051. doi:10.1016/j.jclepro.2021.129051
Qasem, N. A. A., Mohammed, R. H., and Lawal, D. U. (2021). Removal of heavy metal ions from wastewater: A comprehensive and critical review. NPJ Clean. Water 4, 36. doi:10.1038/s41545-021-00127-0
Qian, X., Zhou, J., and Chen, G. (2021). Phonon-engineered extreme thermal conductivity materials. Nat. Mater 20, 1188–1202. doi:10.1038/s41563-021-00918-3
Quan, X., Mei, Y., Xu, H., Sun, B., and Zhang, X. (2015). Optimization of Pt-Pd alloy catalyst and supporting materials for oxygen reduction in air-cathode Microbial Fuel Cells. Electrochim Acta 165, 72–77. doi:10.1016/j.electacta.2015.02.235
Qutub, N., Singh, P., Sabir, S., Sagadevan, S., and Oh, W.-C. (2022). Enhanced photocatalytic degradation of Acid Blue dye using CdS/TiO2 nanocomposite. Sci. Rep. 12, 5759. doi:10.1038/s41598-022-09479-0
Raina, S., Roy, A., and Bharadvaja, N. (2020). Degradation of dyes using biologically synthesized silver and copper nanoparticles. Environ. Nanotechnol. Monit. Manag. 13, 100278. doi:10.1016/j.enmm.2019.100278
Rajabi, H., Jafari, S. M., Feizy, J., Ghorbani, M., and Mohajeri, S. A. (2020). Preparation and characterization of 3D graphene oxide nanostructures embedded with nanocomplexes of chitosan-gum Arabic biopolymers. Int. J. Biol. Macromol. 162, 163–174. doi:10.1016/j.ijbiomac.2020.06.076
Rajabi, M., Mahanpoor, K., and Moradi, O. (2017). Removal of dye molecules from aqueous solution by carbon nanotubes and carbon nanotube functional groups: Critical review. RSC Adv. 7, 47083–47090. doi:10.1039/C7RA09377B
Rajeev, R., Datta, R., Varghese, A., Sudhakar, Y. N., and George, L. (2021). Recent advances in bimetallic based nanostructures: Synthesis and electrochemical sensing applications. Microchem. J. 163, 105910. doi:10.1016/j.microc.2020.105910
Rajendran, S., Inwati, G. K., Yadav, V. K., Choudhary, N., Solanki, M. B., Abdellattif, M. H., et al. (2021). Enriched catalytic activity of TiO2 nanoparticles supported by activated carbon for noxious pollutant elimination. Nanomaterials 11, 2808. doi:10.3390/nano11112808
Rajput, P., Sinha, R. K., and Devi, P. (2021). “Chapter 8 - current scenario of pesticide contamination in water,” in Contamination of water. Editors A. Ahamad, S. I. Siddiqui, and P. Singh (United States: Academic Press), 109–119. doi:10.1016/B978-0-12-824058-8.00032-3
Ramakrishna, S., and Shirazi, A. M. M. (2015). Electrospun membranes: Next generation membranes for desalination and water/wastewater treatment. J. Membr. Sci. Res. 1, 46–47. doi:10.22079/jmsr.2015.12306
Ramani, T., Leon Prasanth, K., and Sreedhar, B. (2016). Air stable colloidal copper nanoparticles: Synthesis, characterization and their surface-enhanced Raman scattering properties. Phys. E Low. Dimens. Syst. Nanostruct 77, 65–71. doi:10.1016/j.physe.2015.11.002
Ramos, M. A. V., Yan, W., Li, X., Koel, B. E., and Zhang, W. (2009). Simultaneous oxidation and reduction of arsenic by zero-valent iron nanoparticles: Understanding the significance of the Core−Shell structure. J. Phys. Chem. C 113, 14591–14594. doi:10.1021/jp9051837
Rao, N., Singh, R., and Bashambu, L. (2021). Carbon-based nanomaterials: Synthesis and prospective applications. Mater Today Proc. 44, 608–614. doi:10.1016/j.matpr.2020.10.593
Rashtbari, Y., Sher, F., Afshin, S., Hamzezadeh, A., Ahmadi, S., Azhar, O., et al. (2022). Green synthesis of zero-valent iron nanoparticles and loading effect on activated carbon for furfural adsorption. Chemosphere 287, 132114. doi:10.1016/j.chemosphere.2021.132114
Raut, A. V., Yadav, H. M., Gnanamani, A., Pushpavanam, S., and Pawar, S. H. (2016). Synthesis and characterization of chitosan-TiO2:Cu nanocomposite and their enhanced antimicrobial activity with visible light. Colloids Surf. B Biointerfaces 148, 566–575. doi:10.1016/j.colsurfb.2016.09.028
Ray, S. S., and Bandyopadhyay, J. (2021). Nanotechnology-enabled biomedical engineering: Current trends, future scopes, and perspectives, Nanotechnol. Rev. 10, 728–743. doi:10.1515/ntrev-2021-0052
Ringu, T., Ghosh, S., Das, A., and Pramanik, N. (2022). Zinc oxide nanoparticles: An excellent biomaterial for bioengineering applications. Emergent Mater 5, 1629–1648. doi:10.1007/s42247-022-00402-x
Robati, D., Mirza, B., Rajabi, M., Moradi, O., Tyagi, I., Agarwal, S., et al. (2016). Removal of hazardous dyes-BR 12 and methyl orange using graphene oxide as an adsorbent from aqueous phase. Chem. Eng. J. 284, 687–697. doi:10.1016/j.cej.2015.08.131
Rocher, V., Siaugue, J.-M., Cabuil, V., and Bee, A. (2008). Removal of organic dyes by magnetic alginate beads. Water Res. 42, 1290–1298. doi:10.1016/j.watres.2007.09.024
Ryu, A., Jeong, S.-W., Jang, A., and Choi, H. (2011). Reduction of highly concentrated nitrate using nanoscale zero-valent iron: Effects of aggregation and catalyst on reactivity. Appl. Catal. B 105, 128–135. doi:10.1016/j.apcatb.2011.04.002
Sadak, M. S., and Bakry, B. A. (2020). Zinc-oxide and nano ZnO oxide effects on growth, some biochemical aspects, yield quantity, and quality of flax (Linum uitatissimum L) in absence and presence of compost under sandy soil. Bull. Natl. Res. Cent. 44, 98. doi:10.1186/s42269-020-00348-2
Saeed, S. M., and Shaker, I. M. (2008). “Assessment of heavy metals pollution in water and sediments and their effect on Oreochromis niloticus in the northern Delta lakes, Egypt,” in 8th international symposium on Tilapia in aquaculture (United States: Academic Press), 475–490.
Sagadevan, S., Fatimah, I., Egbosiub, T. C., Alshahateet, S. F., Lett, J. A., Weldegebrieal, G. K., et al. (2022). Photocatalytic efficiency of titanium dioxide for dyes and heavy metals removal from wastewater. Bull. Chem. React. Eng. Catal. 17, 430–450. doi:10.9767/BCREC.17.2.13948.430-450
Sajid, M., and Płotka-Wasylka, J. (2020). Nanoparticles: Synthesis, characteristics, and applications in analytical and other sciences. Microchem. J. 154, 104623. doi:10.1016/j.microc.2020.104623
Salazar, H., Martins, P. M., Valverde, A., Fernández de Luis, R., Vilas-Vilela, J. L., Ferdov, S., et al. (2022). Reusable nanocomposite membranes for highly efficient arsenite and arsenate dual removal from water. Adv. Mater Interfaces 9, 2101419. doi:10.1002/admi.202101419
Saleem, J., Shahid, U. B., Hijab, M., Mackey, H., and McKay, G. (2019). Production and applications of activated carbons as adsorbents from olive stones. Biomass Convers. Biorefin 9, 775–802. doi:10.1007/s13399-019-00473-7
Saleh, T. A., Mustaqeem, M., and Khaled, M. (2022). Water treatment technologies in removing heavy metal ions from wastewater: A review. Environ. Nanotechnol. Monit. Manag. 17, 100617. doi:10.1016/j.enmm.2021.100617
Scocchi, G., Posocco, P., Fermeglia, M., and Pricl, S. (2007). Polymer−Clay nanocomposites: A multiscale molecular modeling approach. J. Phys. Chem. B 111, 2143–2151. doi:10.1021/jp067649w
Shah, M., Fawcett, D., Sharma, S., Tripathy, S. K., and Poinern, G. E. J. (2015). Green synthesis of metallic nanoparticles via biological entities. Materials 8, 7278–7308. doi:10.3390/ma8115377
Sharma, P., and Das, M. R. (2013). Removal of a cationic dye from aqueous solution using graphene oxide nanosheets: Investigation of adsorption parameters. J. Chem. Eng. Data 58, 151–158. doi:10.1021/je301020n
Sharma, R., Sarkar, A., Jha, R., Sharma, A. K., Bhushan, M., and Bhardwaj, R. (2022). Synthesis & material properties of α-MoO3 nanoparticles. Mater Today Proc. 48, 683–686. doi:10.1016/j.matpr.2021.08.092
Sheela, T., Nayaka, Y. A., Viswanatha, R., Basavanna, S., and Venkatesha, T. G. (2012). Kinetics and thermodynamics studies on the adsorption of Zn(II), Cd(II) and Hg(II) from aqueous solution using zinc oxide nanoparticles. Powder Technol. 217, 163–170. doi:10.1016/j.powtec.2011.10.023
Shehata, N., Egirani, D., Olabi, A. G., Inayat, A., Abdelkareem, M. A., Chae, K.-J., et al. (2023). Membrane-based water and wastewater treatment technologies: Issues, current trends, challenges, and role in achieving sustainable development goals, and circular economy. Chemosphere 320, 137993. doi:10.1016/j.chemosphere.2023.137993
Sheoran, K., Kaur, H., Siwal, S. S., Saini, A. K., Vo, D.-V. N., and Thakur, V. K. (2022). Recent advances of carbon-based nanomaterials (CBNMs) for wastewater treatment: Synthesis and application. Chemosphere 299, 134364. doi:10.1016/j.chemosphere.2022.134364
Shi, Y., Wang, H., Song, G., Zhang, Y., Tong, L., Sun, Y., et al. (2022). Magnetic graphene oxide for methylene blue removal: Adsorption performance and comparison of regeneration methods. Environ. Sci. Pollut. Res. 29, 30774–30789. doi:10.1007/s11356-021-17654-5
Shih, Y., and Tai, Y. (2010). Reaction of decabrominated diphenyl ether by zerovalent iron nanoparticles. Chemosphere 78, 1200–1206. doi:10.1016/j.chemosphere.2009.12.061
Shipley, H. J., Engates, K. E., and Grover, V. A. (2013). Removal of Pb(II), Cd(II), Cu(II), and Zn(II) by hematite nanoparticles: Effect of sorbent concentration, pH, temperature, and exhaustion. Environ. Sci. Pollut. Res. 20, 1727–1736. doi:10.1007/s11356-012-0984-z
Shirsath, D. S., and Shirivastava, V. S. (2015). Adsorptive removal of heavy metals by magnetic nanoadsorbent: An equilibrium and thermodynamic study. Appl. Nanosci. Switz. 5, 927–935. doi:10.1007/s13204-014-0390-6
Singh, H. L., Garg, R., Jana, A., Bathula, C., Naik, S., and Mittal, M. (2023b). Current developments in nanostructurally engineered metal oxide for removal of contaminants in water. Ceram. Int. 49, 7308–7321. doi:10.1016/j.ceramint.2022.10.183
Singh, H. L., Khaturia, S., Chahar, M., and Bishnoi, A. (2023a). Membrane and membrane-based processes for wastewater treatment. United States: CRC Press, 51–65. doi:10.1201/9781003165019-4
Singh, K. K., Arkoti, N. K., Verma, V., and Pal, K. (2022b). “Nanomaterials and their distinguishing features,” in Nanomaterials for advanced technologies. Editors J. K. Katiyar, V. Panwar, and N. Ahlawat (Singapore: Springer Nature Singapore), 1–18. doi:10.1007/978-981-19-1384-6_1
Singh, K. K., Singh, A., and Rai, S. (2022a). A study on nanomaterials for water purification. Mater Today Proc. 51, 1157–1163. doi:10.1016/j.matpr.2021.07.116
Singh, K. R. B., Nayak, V., Sarkar, T., and Singh, R. P. (2020). Cerium oxide nanoparticles: Properties, biosynthesis and biomedical application. RSC Adv. 10, 27194–27214. doi:10.1039/D0RA04736H
Soltani, S., Gacem, A., Choudhary, N., Yadav, V. K., Alsaeedi, H., Modi, S., et al. (2023). Scallion peel mediated synthesis of zinc oxide nanoparticles and their applications as nano fertilizer and photocatalyst for removal of organic pollutants from wastewater. WaterSwitzerl. 15, 1672. doi:10.3390/w15091672
Soyekwo, F., Zhang, Q. G., Deng, C., Gong, Y., Zhu, A. M., and Liu, Q. L. (2014). Highly permeable cellulose acetate nanofibrous composite membranes by freeze-extraction. J. Memb. Sci. 454, 339–345. doi:10.1016/j.memsci.2013.12.014
Sun, H., Liu, S., Zhou, G., Ang, H. M., Tadé, M. O., and Wang, S. (2012). Reduced graphene oxide for catalytic oxidation of aqueous organic pollutants. ACS Appl. Mater Interfaces 4, 5466–5471. doi:10.1021/am301372d
Sun, H., Zhou, Q., Zhao, L., and Wu, W. (2021). Enhanced simultaneous removal of nitrate and phosphate using novel solid carbon source/zero-valent iron composite. J. Clean. Prod. 289, 125757. doi:10.1016/j.jclepro.2020.125757
Sutirman, Z. A., Sanagi, M. M., and Wan Aini, W. I. (2021). Alginate-based adsorbents for removal of metal ions and radionuclides from aqueous solutions: A review. Int. J. Biol. Macromol. 174, 216–228. doi:10.1016/j.ijbiomac.2021.01.150
Tang, F. H. M., Lenzen, M., McBratney, A., and Maggi, F. (2021). Risk of pesticide pollution at the global scale. Nat. Geosci. 14, 206–210. doi:10.1038/s41561-021-00712-5
Tarekegn, M. M., Balakrishnan, R. M., Hiruy, A. M., and Dekebo, A. H. (2021a). Removal of methylene blue dye using nano zerovalent iron, nanoclay and iron impregnated nanoclay – A comparative study. RSC Adv. 11, 30109–30131. doi:10.1039/D1RA03918K
Tarekegn, M. M., Hiruy, A. M., and Dekebo, A. H. (2021b). Nano zero valent iron (nZVI) particles for the removal of heavy metals (Cd2+, Cu2+ and Pb2+) from aqueous solutions. RSC Adv. 11, 18539–18551. doi:10.1039/D1RA01427G
Tavker, N., Yadav, V. K., Yadav, K. K., Cabral-Pinto, M. M. S., Alam, J., Shukla, A. K., et al. (2021). Removal of cadmium and chromium by mixture of silver nanoparticles and nano-fibrillated cellulose isolated from waste peels of citrus sinensis. Polym. (Basel) 13, 234–314. doi:10.3390/polym13020234
Thiruvengadam, M., Rajakumar, G., and Chung, I. M. (2018). Nanotechnology: Current uses and future applications in the food industry. 3 Biotech. 8, 74. doi:10.1007/s13205-018-1104-7
Titchou, F. E., Zazou, H., Afanga, H., El Gaayda, J., Ait Akbour, R., Nidheesh, P. V., et al. (2021). Removal of organic pollutants from wastewater by advanced oxidation processes and its combination with membrane processes. Chem. Eng. Process. - Process Intensif. 169, 108631. doi:10.1016/j.cep.2021.108631
Tiwari, J. N., Mahesh, K., Le, N. H., Kemp, K. C., Timilsina, R., Tiwari, R. N., et al. (2013). Reduced graphene oxide-based hydrogels for the efficient capture of dye pollutants from aqueous solutions. Carbon N. Y. 56, 173–182. doi:10.1016/j.carbon.2013.01.001
Tolcha, T., Gemechu, T., and Megersa, N. (2020). Flower of typha latifolia as a low-cost adsorbent for quantitative uptake of multiclass pesticide residues from contaminated waters. South Afr. J. Chem. 73, 22–29. doi:10.17159/0379-4350/2020/V73A4
Tran, H. V., Bui, L. T., Dinh, T. T., Le, D. H., Huynh, C. D., and Trinh, A. X. (2017). Graphene oxide/Fe3O4/chitosan nanocomposite: A recoverable and recyclable adsorbent for organic dyes removal. Application to methylene blue. Mater Res. Express 4, 035701. doi:10.1088/2053-1591/aa6096
Umehara, M., Kumamoto, Y., Mukai, K., and Isogai, A. (2022). Iron (III) oxyhydroxide powders with TEMPO-oxidized cellulose nanofibrils: Effective adsorbents for removal of fluoride ion in water. Cellulose 29, 9283–9295. doi:10.1007/s10570-022-04842-w
Urian, Y. A., Atoche-Medrano, J. J., Quispe, L. T., León Félix, L., and Coaquira, J. A. H. (2021). Study of the surface properties and particle-particle interactions in oleic acid-coated Fe3O4 nanoparticles. J. Magn. Magn. Mater 525, 167686. doi:10.1016/j.jmmm.2020.167686
Uwamungu, J. Y., Kumar, P., Alkhayyat, A., Younas, T., Capangpangan, R. Y., Alguno, A. C., et al. (2022). Future of water/wastewater treatment and management by industry 4.0 integrated nanocomposite manufacturing. J. Nanomater 2022, 1–11. doi:10.1155/2022/5316228
Van Thuan, D., Nguyen, T. L., Pham Thi, H. H., Thanh, N. T., Ghotekar, S., Sharma, A. K., et al. (2022). Development of Indium vanadate and Silver deposited on graphitic carbon nitride ternary heterojunction for advanced photocatalytic degradation of residual antibiotics in aqueous environment. Opt. Mater (Amst) 123, 111885. doi:10.1016/j.optmat.2021.111885
Vasiliev, G., Kubo, A.-L., Vija, H., Kahru, A., Bondar, D., Karpichev, Y., et al. (2023). Synergistic antibacterial effect of copper and silver nanoparticles and their mechanism of action. Sci. Rep. 13, 9202. doi:10.1038/s41598-023-36460-2
Vasudevan, S., and Lakshmi, J. (2012). The adsorption of phosphate by graphene from aqueous solution. RSC Adv. 2, 5234–5242. doi:10.1039/C2RA20270K
Vatutsina, O. M., Soldatov, V. S., Sokolova, V. I., Johann, J., Bissen, M., and Weissenbacher, A. (2007). A new hybrid (polymer/inorganic) fibrous sorbent for arsenic removal from drinking water. React. Funct. Polym. 67, 184–201. doi:10.1016/j.reactfunctpolym.2006.10.009
Veeman, D., Shree, M. V., Sureshkumar, P., Jagadeesha, T., Natrayan, L., Ravichandran, M., et al. (2021). Sustainable development of carbon nanocomposites: Synthesis and classification for environmental remediation. J. Nanomater 2021, 1–21. doi:10.1155/2021/5840645
Villalva, M. D., Agarwal, V., Ulanova, M., Sachdev, P. S., and Braidy, N. (2021). Quantum dots as a theranostic approach in alzheimer’s disease: A systematic review. Nanomedicine 16, 1595–1611. doi:10.2217/nnm-2021-0104
Vu, X. H., Dien, N. D., Ha Pham, T. T., Trang, T. T., Ca, N. X., Tho, P. T., et al. (2020). The sensitive detection of methylene blue using silver nanodecahedra prepared through a photochemical route. RSC Adv. 10, 38974–38988. doi:10.1039/d0ra07869g
Wang, H., Hu, B., Gao, Z., Zhang, F., and Wang, J. (2021). Emerging role of graphene oxide as sorbent for pesticides adsorption: Experimental observations analyzed by molecular modeling. J. Mater Sci. Technol. 63, 192–202. doi:10.1016/j.jmst.2020.02.033
Waris, A., Din, M., Ali, A., Ali, M., Afridi, S., Baset, A., et al. (2021). A comprehensive review of green synthesis of copper oxide nanoparticles and their diverse biomedical applications. Inorg. Chem. Commun. 123, 108369. doi:10.1016/j.inoche.2020.108369
Wei, H., Rodriguez, K., Renneckar, S., Leng, W., and Vikesland, P. J. (2015). Preparation and evaluation of nanocellulose–gold nanoparticle nanocomposites for SERS applications. Analyst 140, 5640–5649. doi:10.1039/C5AN00606F
Wei, X., Bhojappa, S., Lin, L.-S., and Viadero, R. C. (2011). Performance of nano-magnetite for removal of selenium from aqueous solutions. Environ. Eng. Sci. 29, 526–532. doi:10.1089/ees.2011.0383
Wen, Z., Zhang, Y., and Dai, C. (2014). Removal of phosphate from aqueous solution using nanoscale zerovalent iron (nZVI). Colloids Surf. A Physicochem Eng. Asp. 457, 433–440. doi:10.1016/j.colsurfa.2014.06.017
Witkowska, D., Słowik, J., and Chilicka, K. (2021). Heavy metals and human health: Possible exposure pathways and the competition for protein binding sites. Molecules 26, 6060. doi:10.3390/molecules26196060
Wu, L., Li, M., Li, M., Sun, Q., and Zhang, C. (2020). Preparation of RGO and anionic polyacrylamide composites for removal of Pb(II) in aqueous solution. Polym. (Basel) 12, 1426. doi:10.3390/polym12061426
Xiu, Z.-M., Ma, J., and Alvarez, P. J. J. (2011). Differential effect of common ligands and molecular oxygen on antimicrobial activity of silver nanoparticles versus silver ions. Environ. Sci. Technol. 45, 9003–9008. doi:10.1021/es201918f
Xu, L., and Wang, J. (2017). The application of graphene-based materials for the removal of heavy metals and radionuclides from water and wastewater. Crit. Rev. Environ. Sci. Technol. 47, 1042–1105. doi:10.1080/10643389.2017.1342514
Xu, P., Zeng, G. M., Huang, D. L., Feng, C. L., Hu, S., Zhao, M. H., et al. (2012). Use of iron oxide nanomaterials in wastewater treatment: A review. Sci. Total Environ. 424, 1–10. doi:10.1016/j.scitotenv.2012.02.023
Yadav, V. K., Amari, A., Gacem, A., Elboughdiri, N., Eltayeb, L. B., and Fulekar, M. H. (2023a). Treatment of fly-ash-contaminated wastewater loaded with heavy metals by using fly-ash-synthesized iron oxide nanoparticles. WaterSwitzerl. 15, 908. doi:10.3390/w15050908
Yadav, V. K., Amari, A., Wanale, S. G., Osman, H., and Fulekar, M. H. (2023b). Synthesis of floral-shaped nanosilica from coal fly ash and its application for the remediation of heavy metals from fly ash aqueous solutions. Sustainability 15, 2612. doi:10.3390/su15032612
Yadav, V. K., and Fulekar, M. H. (2018). Biogenic synthesis of maghemite nanoparticles (γ-Fe2O3) using Tridax leaf extract and its application for removal of fly ash heavy metals (Pb, Cd). Mater Today Proc. 5, 20704–20710. doi:10.1016/j.matpr.2018.06.454
Yadav, V. K., Gnanamoorthy, G., Ali, D., Bera, S. P., Roy, A., Kumar, G., et al. (2022a). Cytotoxicity, removal of Congo red dye in aqueous solution using synthesized amorphous iron oxide nanoparticles from incense sticks ash waste. J. Nanomater 2022, 1–12. doi:10.1155/2022/5949595
Yadav, V. K., Yadav, K. K., Gacem, A., Gnanamoorthy, G., Ali, I. H., Khan, S. H., et al. (2022b). A novel approach for the synthesis of vaterite and calcite from incense sticks ash waste and their potential for remediation of dyes from aqueous solution. Sustain Chem. Pharm. 29, 100756. doi:10.1016/j.scp.2022.100756
Yang, J., Shojaei, S., and Shojaei, S. (2022). Removal of drug and dye from aqueous solutions by graphene oxide: Adsorption studies and chemometrics methods. NPJ Clean. Water 5, 5. doi:10.1038/s41545-022-00148-3
Yang, W., Du, X., Zhao, J., Chen, Z., Li, J., Xie, J., et al. (2020). Hydrated eutectic electrolytes with ligand-oriented solvation shells for long-cycling zinc-organic batteries. Joule 4, 1557–1574. doi:10.1016/j.joule.2020.05.018
Yang, Y., Wang, H., Li, J., He, B., Wang, T., and Liao, S. (2012). Novel functionalized nano-TiO2 loading electrocatalytic membrane for oily wastewater treatment. Environ. Sci. Technol. 46, 6815–6821. doi:10.1021/es3000504
Yilmaz, M., Al-Musawi, T. J., Saloot, M., Khatibi, A. D., Baniasadi, M., and Balarak, D. (2022). Synthesis of activated carbon from Lemna minor plant and magnetized with iron (III) oxide magnetic nanoparticles and its application in removal of Ciprofloxacin. Biomass Convers. Biorefin 21, 02279. doi:10.1007/s13399-021-02279-y
Yu, R.-F., Chi, F.-H., Cheng, W.-P., and Chang, J.-C. (2014). Application of pH, ORP, and DO monitoring to evaluate chromium(VI) removal from wastewater by the nanoscale zero-valent iron (nZVI) process. Chem. Eng. J. 255, 568–576. doi:10.1016/j.cej.2014.06.002
Zanata, L., Tofanello, A., Martinho, H. S., Souza, J. A., and Rosa, D. S. (2022). Iron oxide nanoparticles–cellulose: A comprehensive insight on nanoclusters formation. J. Mater Sci. 57, 324–335. doi:10.1007/s10853-021-06564-z
Zhang, J., Niu, Y., Zhou, Y., Ju, S., and Gu, Y. (2022). Green preparation of nano-zero-valent iron-copper bimetals for nitrate removal: Characterization, reduction reaction pathway, and mechanisms. Adv. Powder Technol. 33, 103807. doi:10.1016/j.apt.2022.103807
Zhang, L., Shao, Q., and Xu, C. (2019). Enhanced azo dye removal from wastewater by coupling sulfidated zero-valent iron with a chelator. J. Clean. Prod. 213, 753–761. doi:10.1016/j.jclepro.2018.12.183
Zhang, M., Gao, B., Yao, Y., Xue, Y., and Inyang, M. (2012). Synthesis of porous MgO-biochar nanocomposites for removal of phosphate and nitrate from aqueous solutions. Chem. Eng. J. 210, 26–32. doi:10.1016/j.cej.2012.08.052
Zhang, Q., Pan, B., Pan, B., Zhang, W., Jia, K., and Zhang, Q. (2008). Selective sorption of lead, cadmium and zinc ions by a polymeric cation exchanger containing nano-Zr(HPO3S)2. Environ. Sci. Technol. 42, 4140–4145. doi:10.1021/es800354b
Zhang, Q., Pan, B., Zhang, S., Wang, J., Zhang, W., and Lv, L. (2011a). New insights into nanocomposite adsorbents for water treatment: A case study of polystyrene-supported zirconium phosphate nanoparticles for lead removal. J. Nanoparticle Res. 13, 5355–5364. doi:10.1007/s11051-011-0521-x
Zhang, Q., Shao, Y., Liu, J., Aksay, I. A., and Lin, Y. (2011b). Graphene-polypyrrole nanocomposite as a highly efficient and low cost electrically switched ion exchanger for removing ClO4- from wastewater. ACS Appl. Mater Interfaces 3, 3633–3637. doi:10.1021/am200839m
Zhao, B., Xu, X., Zhang, R., and Cui, M. (2021). Remediation of Cu(II) and its adsorption mechanism in aqueous system by novel magnetic biochar derived from co-pyrolysis of sewage sludge and biomass. Environ. Sci. Pollut. Res. 28, 16408–16419. doi:10.1007/s11356-020-11811-y
Zhao, X., Lv, L., Pan, B., Zhang, W., Zhang, S., and Zhang, Q. (2011). Polymer-supported nanocomposites for environmental application: A review. Chem. Eng. J. 170, 381–394. doi:10.1016/j.cej.2011.02.071
Zhao, X., Song, N., Jia, Q., and Zhou, W. (2009). Determination of Cu, Zn, Mn, and Pb by microcolumn packed with multiwalled carbon nanotubes on-line coupled with flame atomic absorption spectrometry. Microchim. Acta 166, 329–335. doi:10.1007/s00604-009-0204-9
Zhou, Q., Dong, Z.-Y., Hsieh, W.-P., Goncharov, A. F., and Chen, X.-J. (2022b). Thermal conductivity of materials under pressure. Nat. Rev. Phys. 4, 319–335. doi:10.1038/s42254-022-00423-9
Zhou, Q., Sun, H., Jia, L., Wu, W., and Wang, J. (2022a). Simultaneous biological removal of nitrogen and phosphorus from secondary effluent of wastewater treatment plants by advanced treatment: A review. Chemosphere 296, 134054. doi:10.1016/j.chemosphere.2022.134054
Zhou, Y., Wang, T., Zhi, D., Guo, B., Zhou, Y., Nie, J., et al. (2019). Applications of nanoscale zero-valent iron and its composites to the removal of antibiotics: A review. J. Mater Sci. 54, 12171–12188. doi:10.1007/s10853-019-03606-5
Zhu, H., Chen, D., Yang, S., Li, N., Xu, Q., Li, H., et al. (2016). A versatile and cost-effective reduced graphene oxide-crosslinked polyurethane sponge for highly effective wastewater treatment. RSC Adv. 6, 38350–38355. doi:10.1039/C6RA05450A
Zhu, Q., and Li, Z. (2015). Hydrogel-supported nanosized hydrous manganese dioxide: Synthesis, characterization, and adsorption behavior study for Pb2+, Cu2+, Cd2+ and Ni2+ removal from water. Chem. Eng. J. 281, 69–80. doi:10.1016/j.cej.2015.06.068
Zhu, S., Wang, X., Cong, Y., and Li, L. (2020). Regulating the optical properties of gold nanoclusters for biological applications. ACS Omega 5, 22702–22707. doi:10.1021/acsomega.0c03218
Zouboulis, A. I., and Katsoyiannis, I. A. (2002). Arsenic removal using iron oxide loaded alginate beads. Ind. Eng. Chem. Res. 41, 6149–6155. doi:10.1021/ie0203835
Keywords: carbon nanotubes, graphene, nanocomposites, nanomaterials, wastewater
Citation: Chahar M, Khaturia S, Singh HL, Solanki VS, Agarwal N, Sahoo DK, Yadav VK and Patel A (2023) Recent advances in the effective removal of hazardous pollutants from wastewater by using nanomaterials—A review. Front. Environ. Sci. 11:1226101. doi: 10.3389/fenvs.2023.1226101
Received: 20 May 2023; Accepted: 25 July 2023;
Published: 04 August 2023.
Edited by:
Spyros Foteinis, Heriot-Watt University, United KingdomReviewed by:
Titus Egbosiuba, Chukwuemeka Odumegwu Ojukwu University, NigeriaSiroos Shojaei, University of Sistan and Baluchestan, Iran
Copyright © 2023 Chahar, Khaturia, Singh, Solanki, Agarwal, Sahoo, Yadav and Patel. This is an open-access article distributed under the terms of the Creative Commons Attribution License (CC BY). The use, distribution or reproduction in other forums is permitted, provided the original author(s) and the copyright owner(s) are credited and that the original publication in this journal is cited, in accordance with accepted academic practice. No use, distribution or reproduction is permitted which does not comply with these terms.
*Correspondence: Sarita Khaturia, saritakhaturia.slas@modyuniversity.ac.in; Vijendra Singh Solanki, vijendrasingh0018@gmail.com; Dipak Kumar Sahoo, dsahoo@iastate.edu; Ashish Patel, uni.ashish@gmail.com