- 1Leibniz Institute for Prevention Research and Epidemiology—BIPS, Department Epidemiological Methods and Etiological Research, Bremen, Germany
- 2Leibniz Institute for Prevention Research and Epidemiology—BIPS, Library, Bremen, Germany
- 3Leibniz Institute for Prevention Research and Epidemiology—BIPS, Department Clinical Epidemiology, Bremen, Germany
- 4University of Bremen, Faculty of Human and Health Sciences, Bremen, Germany
- 5University of Bremen, Faculty of Mathematics and Computer Science, Bremen, Germany
Introduction: Infections may play a role in the etiology of childhood cancer and immunizations may be protective because vaccinations stimulate the immune system. Observational studies reported inconsistent associations between vaccination and risk of childhood cancer. Since a synthesis of the evidence is lacking, we conducted a meta-analysis stratified by histological and site-specific cancer.
Methods: We performed a systematic review (CRD42020148579) following PRISMA guidelines and searched for literature in MEDLINE, Embase, and the Science Citation Index databases. We identified in three literature databases 7,594 different articles of which 35 met the inclusion criteria allowing for 27 analyses of 11 cancer outcomes after exposure to nine different types of vaccinations. We calculated summary odds ratios (ORs) and 95% confidence intervals (CIs) using random effects models.
Results: We observed four inverse associations between childhood leukemia and certain vaccines as well as the number of vaccinations: OR 0.49 (95% CI = 0.32 to 0.74) for leukemia death after bacillus Calmette–Guérin vaccination; OR 0.76 (95% CI = 0.65 to 0.90) for acute lymphoblastic leukemia after Haemophilus influenzae type b vaccination; OR 0.57 (95% CI = 0.36 to 0.88) for leukemia; and OR 0.62 (95% CI = 0.46 to 0.85) for acute lymphoblastic leukemia after three or more vaccinations of any type. All other conducted analyses did not show any associations.
Discussion: The results are consistent with the hypothesis that vaccinations reduce the risk of childhood leukemia. However, the robustness and validity of these results is limited due to the small number, substantial heterogeneity, and methodological limitations of available studies.
Introduction
Childhood cancers include a broad spectrum of histological and site-specific cancers occurring before 18 years of age (1–3). An estimated 10% of childhood cancers can be traced back to specific rare genetic syndromes with a high cancer risk (4, 5) or a common genetic susceptibility with a small increased risk for childhood cancer (6–11). The only established environmental risk factors are high doses of ionizing radiation (3) and certain chemicals such as benzene for leukemia (12) and for acute myeloid leukemia (AML) cytostatic drugs (5). However, the evidence to date does not suggest that environmental risk factors alone can explain the majority of childhood cancers. Indeed gene-environment interactions of several pre- and postnatal factors are assumed to be involved in their etiology (3, 13).
The peaks in the incidence of acute leukemia in children aged 2 to 5 years that parallel the peaks in infection rates supports the hypothesis that immunological risk factors are involved in the etiology of leukemia (14–16). However, no single infectious agent has been identified as a risk factor for the development of leukemia to date (2, 17). Instead, Kinlen (18–20) proposed the “population mixing” hypothesis with an increased risk for leukemia and non-Hodgkin lymphoma in isolated areas due to lower herd immunity to infections. In addition, Greaves (21) suggested the “delayed infection” hypothesis with a higher risk of acute lymphoblastic leukemia (ALL) in children who did not experience any strengthening of the immune system by an acquired infection in their first year of life. This theory corresponds to the current state of science according to which the immune system plays an important role in the development of cancer (22). For acute leukemia, the possible protective role of immunization is based on the assumption that vaccines also stimulate a better performance of the immune system by formation of antibodies (23). Moreover, it has been suggested that vaccination regulates the risk of childhood cancer in general by non-specific stimulation of certain macrophages and natural killer cells that target tumors (24). This non-specific effects of vaccines may be related to cross-reactivity of the adaptive immune system with unrelated pathogens and to training of the innate immune system through epigenetic reprogramming (25). However, the beneficial effect may be limited to specific vaccines e.g. live vaccines, may be reversed with other vaccines and thus may depend on the sequence of different vaccinations (25). In line with these theories, epidemiological studies investigated the relationship between various factors (26–31) that could stimulate the immune system such as vaccinations and the occurrence of leukemia (32) and other childhood cancers (3, 33).
To summarize evidence on the association between vaccination and childhood cancer, only two meta-analyses have been conducted so far. One focusing on poliomyelitis vaccines, simian virus 40 and human cancer was published in 2004 (34) and another one focusing on early vaccination and childhood leukemia was published in 2017 (32). However, to our knowledge, there is no meta-analysis on different types of vaccinations and the risk of childhood cancer in general or on histological and site-specific subtypes other than leukemia. We aimed to fill this gap and conducted a systematic literature search and meta-analysis of the association between different types of vaccination and the risk of childhood cancer including stratification by cancer sites.
Materials and Methods
Following the meta-analysis of observational studies in epidemiology (MOOSE) guidelines (35) and the preferred reporting items for systematic reviews and meta-analyses (PRISMA) (36), we conducted a comprehensive review of the literature to identify all available risk estimates on the association between vaccination and childhood cancer (PROSPERO registration: CRD42020148579).
Search Strategy
To identify studies on the association between vaccination and childhood cancer, we systematically searched the literature databases MEDLINE, Embase, and the Science Citation Index for relevant articles published before November 2020. We used subject headings and keywords in English depending on the search structure of the literature database to combine the references related to the population, the exposure, and the disease. The search was not restricted by language filters and no date limits or other filters were used. A detailed description of the search strategy is provided in Figure 1. Included articles were also manually searched for potentially relevant citations not detected by the electronic search.
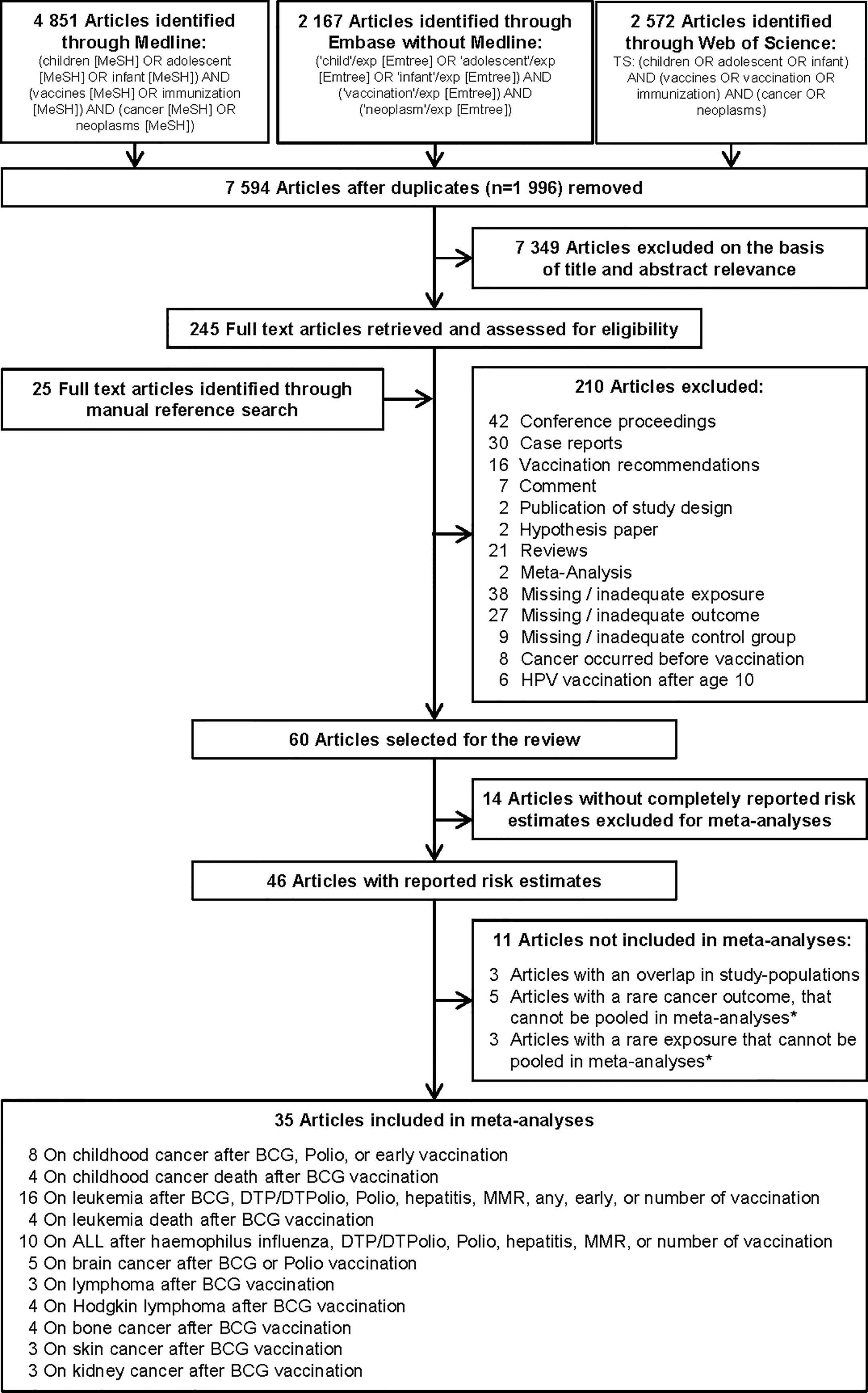
Figure 1 Selection of studies included in the systematic review and meta-analysis on vaccination and risk of childhood cancer. * Studies could not be pooled in the meta-analysis due to an insufficient number of estimates (only one or two) on the same association. ALL, acute lymphoblastic leukemia; BCG, Bacillus Calmette-Guérin; DTP/DTPolio, diphtheria-tetanus-pertussis/-poliomyelitis; MMR, mumps-measles-rubella; Polio, poliomyelitis.
Study Selection
Duplicates found by the three literature searches were deleted using EndNote. Two independent researchers performed the screening of titles and abstracts for relevant publications and conducted the full-text review of selected articles. Studies were considered for inclusion in the review if they met the following three criteria: They were 1) an original epidemiological study that examined the influence of vaccination on the risk of childhood cancer or cancer death; 2) a proper reference group without cancer or without the same cancer as the investigated outcome; and 3) the recommended first application of the studied vaccine should be before age 10 (e.g. exclusion of human papillomaviruses vaccination). Detailed exclusion criteria are shown in Figure 1. For inclusion in the meta-analysis, studies had to report quantitative risk estimates [odds ratio (OR), relative risk (RR), or hazard ratio (HR)], and their variability [variance, standard error (SE), standard deviation (SD), or confidence interval (CI)], or provide the numbers of cases and controls so that crude risk estimates with CI could be calculated. When multiple articles reported on the same population, the most recent article or the most informative publication was included. Since most studies used non-vaccinated children as a reference, we excluded four studies that compared vaccinated children in one time period with vaccinated children in another time period (33, 37–39). Disagreements between the two reviewers were resolved by discussion with a third reviewer.
Data Extraction
Two reviewers extracted a predetermined set of data for each risk estimate on vaccinations and childhood cancer independently from each publication (Supplementary Tables 1 and 2A–C). The extracted data included the following information: name of the first author, year of publication, study location, study period, study design, number of cases (cancers or cancer deaths), number, and type of subjects in the reference group (e.g. population-based or hospital-based), assessment of the outcome, cancer site, age range at diagnosis or death, exposure assessment, vaccine, age at vaccination, matching factors, adjustment variables, reason for exclusion from meta-analysis (no or incomplete risk estimates, i.e. a RR without the 95% CI, without the P value for measures of association and without the number of cases and controls, overlap with other study, less than three studies on an outcome or an exposure), statistical model, risk estimate [OR, RR, HR, standard incidence ratio (SIR) along with SE, SD, 95% CI, P value for measures of association], and number of subjects per group. A third reviewer adjudicated inconsistencies between the two original reviewers.
Quality Assessment
The quality of each study was assessed by applying the Newcastle-Ottawa Scale (NOS) (40), which has been widely used as recommended by the Cochrane Collaboration (41). The NOS comprises nine items categorized into three sections. Depending on the study design, NOS items differ. For case-control studies, the quality was assessed by case definition and representativeness, selection and definition of controls, comparability between cases and controls, ascertainment of exposure, same method of ascertainment for cases and controls, and response proportion. The cohort studies were evaluated for representativeness of the exposure cohort, selection of the non-exposed cohort, ascertainment of exposure, demonstration that outcome of interest was not present at start of study, comparability of cohorts on the basis of the design or analysis, validity of the outcome assessment, duration of follow-up long enough for outcomes to occur, and adequacy of the follow-up cohort (complete follow-up or follow-up >50%). Since the NOS is very general (42) and no other tool for quality assessment is established for observational studies (43), we applied a self-developed scoring system in addition. This score (maximum 45 points) was composed of the following eight criteria, each of which consists of 4 to 15 items: study design (up to six points), study size (up to six points), outcome assessment (up to six points), exposure assessment (up to six points), controlling for confounders (up to six points), statistical methods (up to six points), other methods (up to six points), and reported important characteristics of the study population (up to three points). At the end of the evaluation, there was the possibility to decrease the score by up to six points for selection problems, confounding, bias, and other limitations not described in the eight items before. Quality criteria are detailed in Supplementary Tables 3A, B. Two reviewers extracted the quality items of the included studies independently, discussed the results, and solved disagreements with a third investigator.
Data Analysis
Pooled ORs with 95% CI were calculated using random effects models if three or more studies on a specific research question were available (44). To assess the association of cancer with increasing number of vaccinations (dose-response), trend analyses were conducted based on the method of Greenland and Longnecker (45) where possible. To conduct the trend analyses required for these analyses, at least two estimates with different numbers of vaccination and the same cancer outcome had to be available. The I² statistic was calculated to quantify between-study heterogeneity. We considered values of 50% or less, more than 50 to 75%, and more than 75% to indicate low, moderate, and substantial heterogeneity, respectively (46). Statistical significance of I2 was analyzed with the Q statistic [P value for heterogeneity (P)]. We explored whether heterogeneity could be reduced by omitting each study in turn from the meta-analysis (47). Potential sources of heterogeneity were investigated by conducting subgroup analyses by time period before and after contamination of the poliomyelitis vaccine by carcinogenic simian virus 40 (<1964; 1964+), study design (case-control or ecological study; cohort or case-cohort study), quality of exposure assessment (low: self-report or vaccination card; high: trial, registry, or medical documentation), quality of confounder control (low: basic or no adjustment/matching; high: adjustment or matching for other vaccines or immunological factors), consideration of a latency period (no; yes), Newcastle-Ottawa Scale below and above the fourth quintile (low: quality scale <6; high: quality scale 6+), quality score below and above the fourth quintile (low: detailed quality score <24.7; high: detailed quality score 24.7+), and assessment of outcome via registries (yes: via registries; no: other sources). For analyses with five or more included studies, publication bias was evaluated using funnel plots and the tests described by Egger et al. (48). All P values are two-sided. All calculations were performed using STATA version 14 (StataCorp LP, College Station, TX, USA) (49) or Excel version 2013 (Microsoft Cooperation, Redmond, WA, USA).
Results
Literature Search
Of the 9,590 identified articles, 1,996 were duplicate search results from the three literature databases and 7,349 articles were excluded on the basis of title and abstract screening, leaving 245 articles for full-text evaluation (Figure 1). We identified additional 25 potentially relevant articles by evaluating cross-references. Finally, 210 full-text articles were discarded according to the exclusion criteria, leaving 60 studies (50–109) in the systematic review (Supplementary Table 1). These studies reported 709 risk estimates for different childhood cancers after diverse types of vaccination (Supplementary Tables 2A–C). Overall, 85 effect estimates showed a decreased risk of different childhood cancers, 48 showed an increased risk, and 576 revealed no significant association. For the analyses, 25 of the 60 studies were excluded because (a) they reported no or incomplete risk estimates [number of studies (N) = 14], (b) the study sample overlapped with another included study (N = 3), or (c) the outcome (N = 5) or specific type of vaccination (N = 3) was reported by less than three studies. This left 35 remaining studies for inclusion in 27 specific analyses on 11 different childhood cancer outcomes after exposure to nine different types of vaccination (Figure 1).
Study Characteristics and Quality
Characteristics of the 35 studies included in the meta-analysis are provided in Tables 1–4. Studies were published between 1968 and 2019 and covered a study period of 65 years (1943 to 2008). They were conducted in Europe (57%), North America (26%), South America (8%), Australia or New Zealand (6%), and Asia (3%) with sample sizes ranging from 148 to 1,224,914 participants. Most studies examined only children under 18 years of age, with some exceptions (60, 63, 64, 66, 73, 74, 77, 79, 98, 99, 104). The distribution of selected quality-related factors is summarized in Supplementary Tables 3A, B. The minority of the studies included in the meta-analysis were retrospective cohort or case cohort studies (31%), the majority were case-control studies (69%), of which 18 included population-based (63, 65, 70, 74, 76, 81, 82, 92–95, 97–101, 103, 109) and four hospital-based controls (53, 83, 86, 91). There was only one study with an ecological design that used aggregated data (105). Most studies used laboratory, trial, accounting, registry, or medical documentation to assess the outcome (86%). Only three studies (62, 66, 76) used death certificates, and two studies (77, 94) did not report on this issue. To assess the type and date of vaccination, 40% of the studies used trial, accounting, registry, or medical data, and 40% used parental reports (74, 76, 81–83, 86, 91, 92, 94, 95, 97–99, 109). Further 17% used vaccination cards (63, 93, 100, 101, 103, 104) and 3% used aggregated, external data (105). The majority of studies controlled only for basic confounders (57%), mainly age and sex, while 20% did not take any confounding into account. Ten studies (29%) accounted for a latency period of at least 1 month between the vaccination and the onset of the childhood cancer and verified by this the correct temporal sequence of exposure and outcome. None of the studies reported on the inclusion of secondary cancers and 15 studies (43%) limited their cancers to incidence cases (Supplementary Table 3B). Overall, the methodological quality assessments of the 35 studies included in the meta-analysis yielded an average score of 4.7 out of 9.0 for the NOS and 22.0 out of 45.0 for our own detailed quality score (Supplementary Tables 3A, B). The quality of the 25 studies that were excluded from the meta-analysis was low (mean: 3.8 out of 9.0 points for the NOS and 13.5 out of 45.0 points for the detailed quality score, Supplementary Tables 3A, B). Their characteristics and main results are briefly described in Supplementary Table 1.
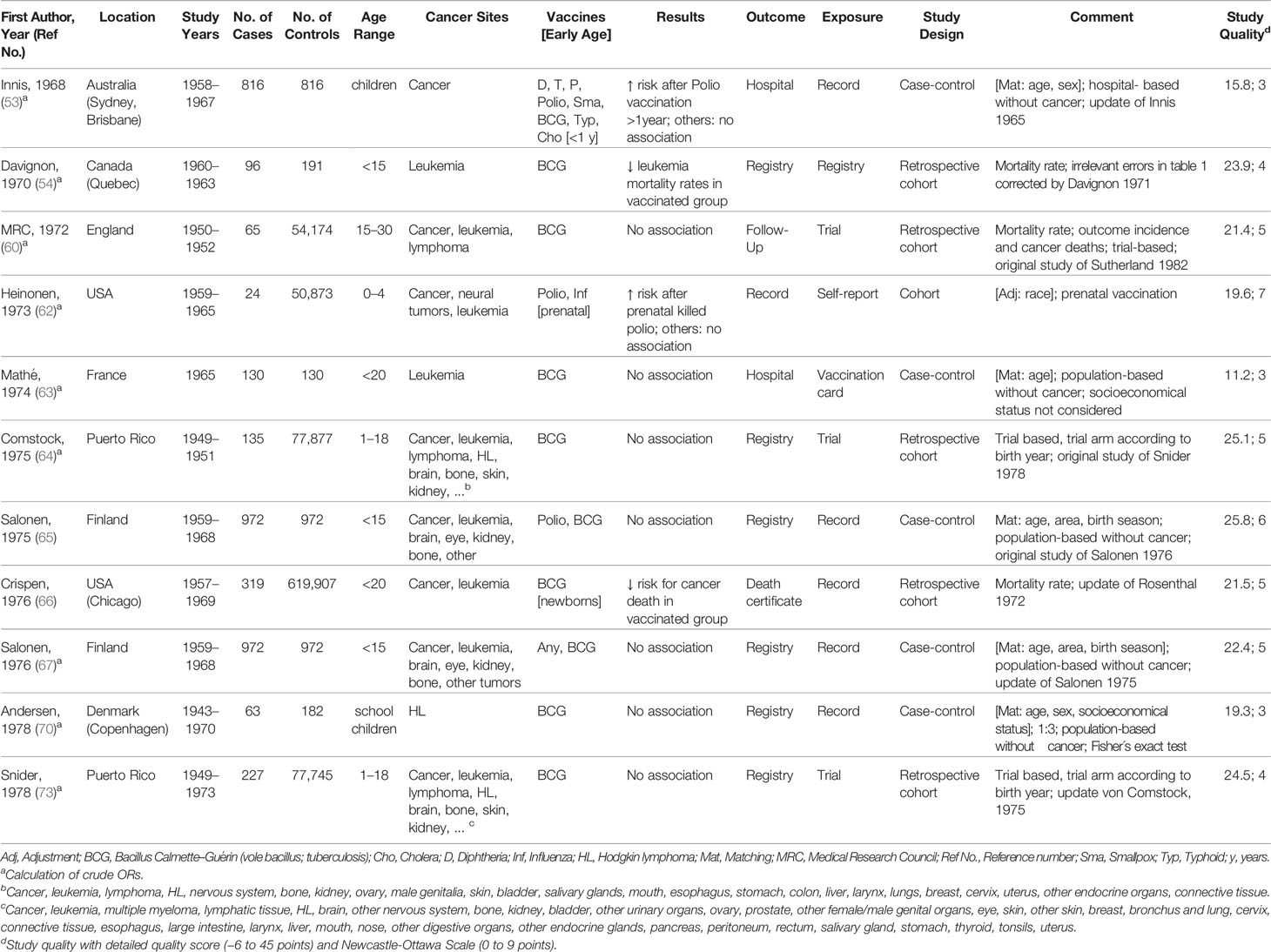
Table 1 Characteristics of studies included in meta-analysis of the associations between vaccination and childhood cancer, 1963–1978.
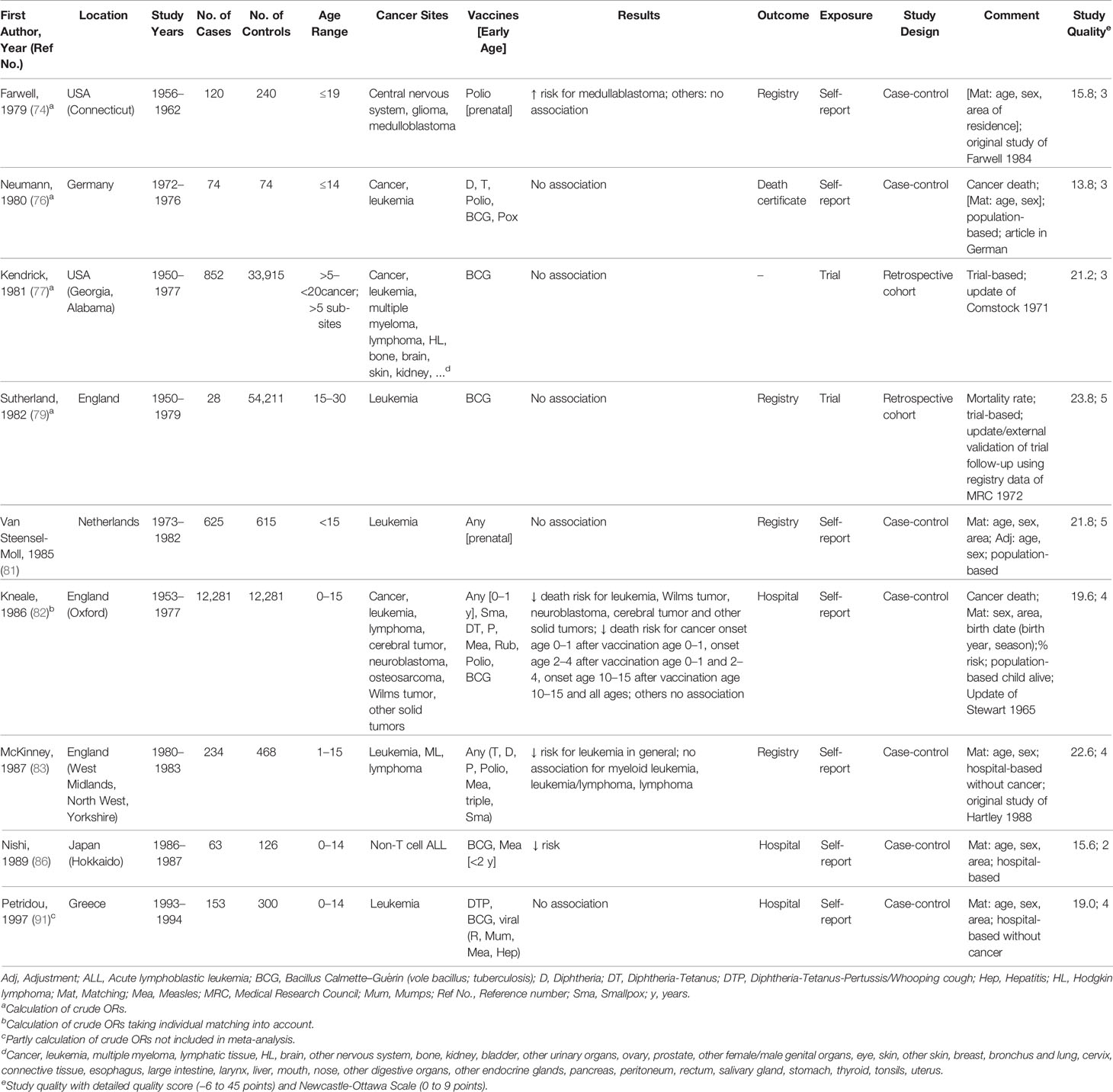
Table 2 Characteristics of studies included in meta-analysis of the associations between vaccination and childhood cancer, 1979–1997.
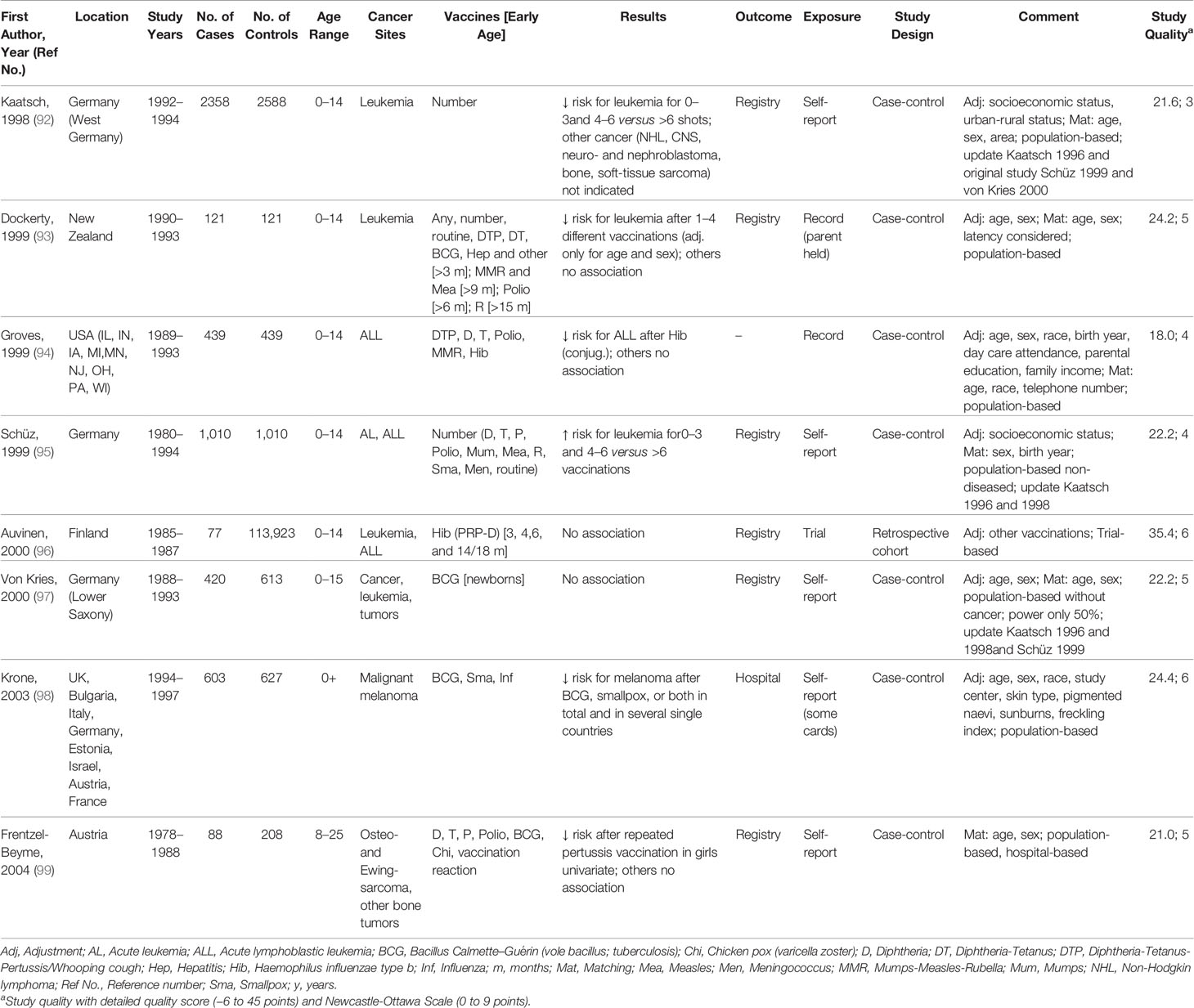
Table 3 Characteristics of studies included in meta-analysis of the associations between vaccination and childhood cancer, 1998–2004.
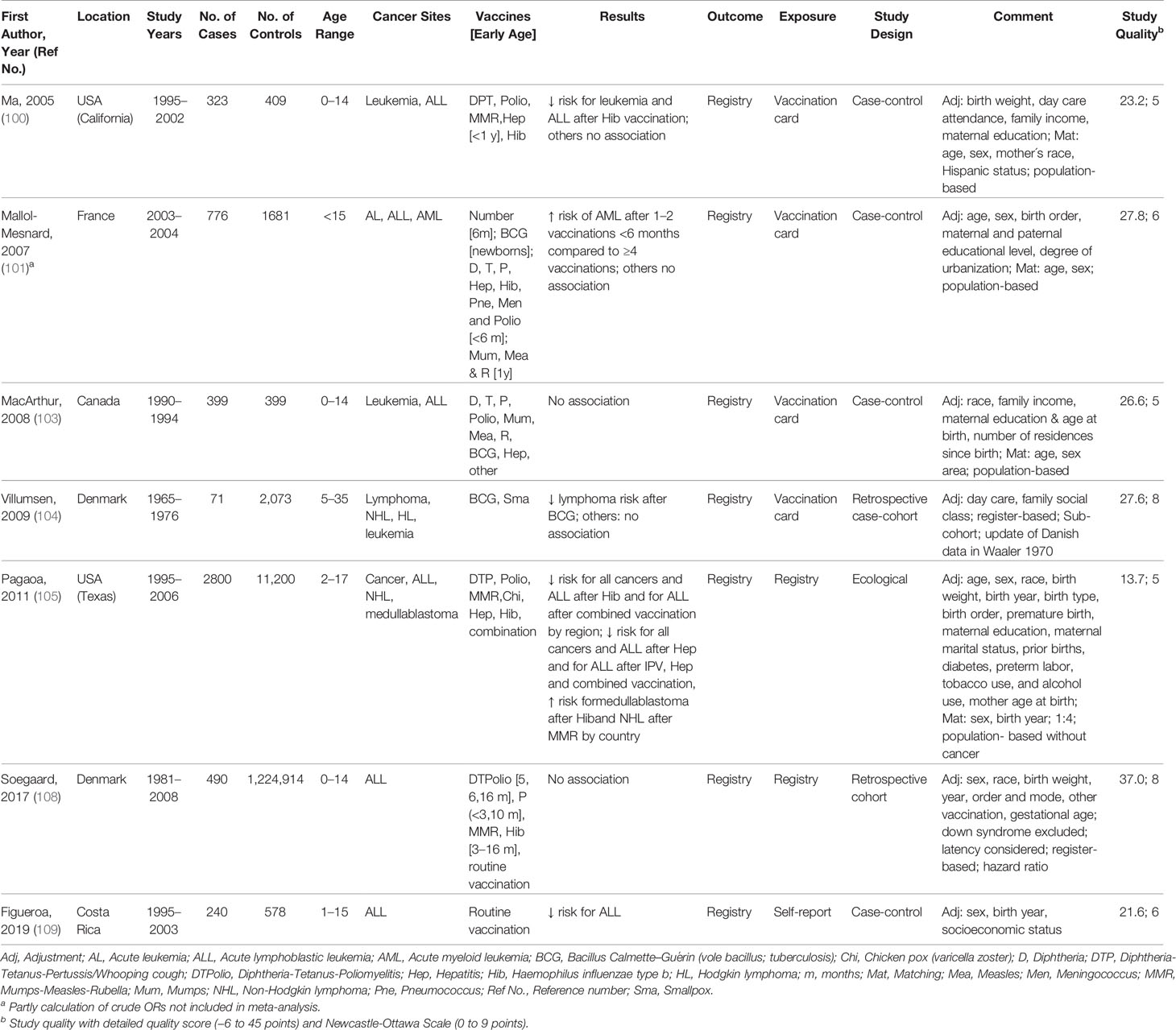
Table 4 Characteristics of studies included in meta-analysis of the associations between vaccination and childhood cancer, 2005–2019.
Results of the Meta-Analysis
Among 27 specific analyses on 11 different childhood cancer outcomes after exposure to nine different types of vaccinations (Figures 2–4), we observed four inverse associations between childhood leukemia and certain vaccines as well as after three or more vaccinations of any type.
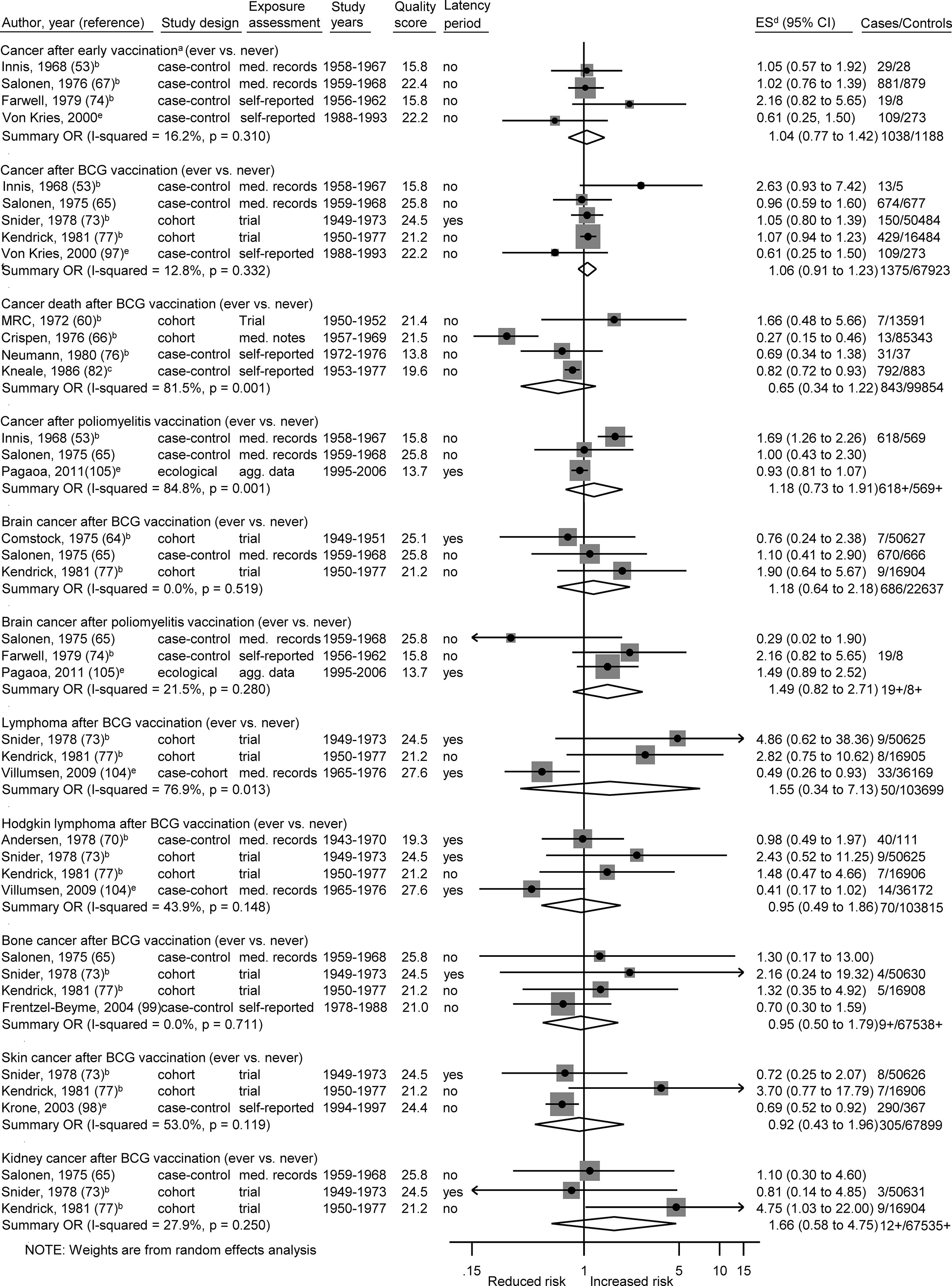
Figure 2 Vaccination and the risk of cancer. agg. data, aggregated data; BCG, Bacillus Calmette-Guérin; med. records, medical records; OR, odds ratio; ES, estimate. (a) Early vaccinations: Innis (poliomyelitis vaccination, age <1), Salonen (any vaccination, perinatal), Farwell (poliomyelitis vaccination, prenatal), von Kries (BCG vaccination, newborns). (b) Calculation of crude ORs. (c) Calculation of crude ORs taking individual matching into account. (d) ES includes single-study odds ratios or hazard ratios and summary odds ratios. (e) Adjusted estimate as indicated by published study.
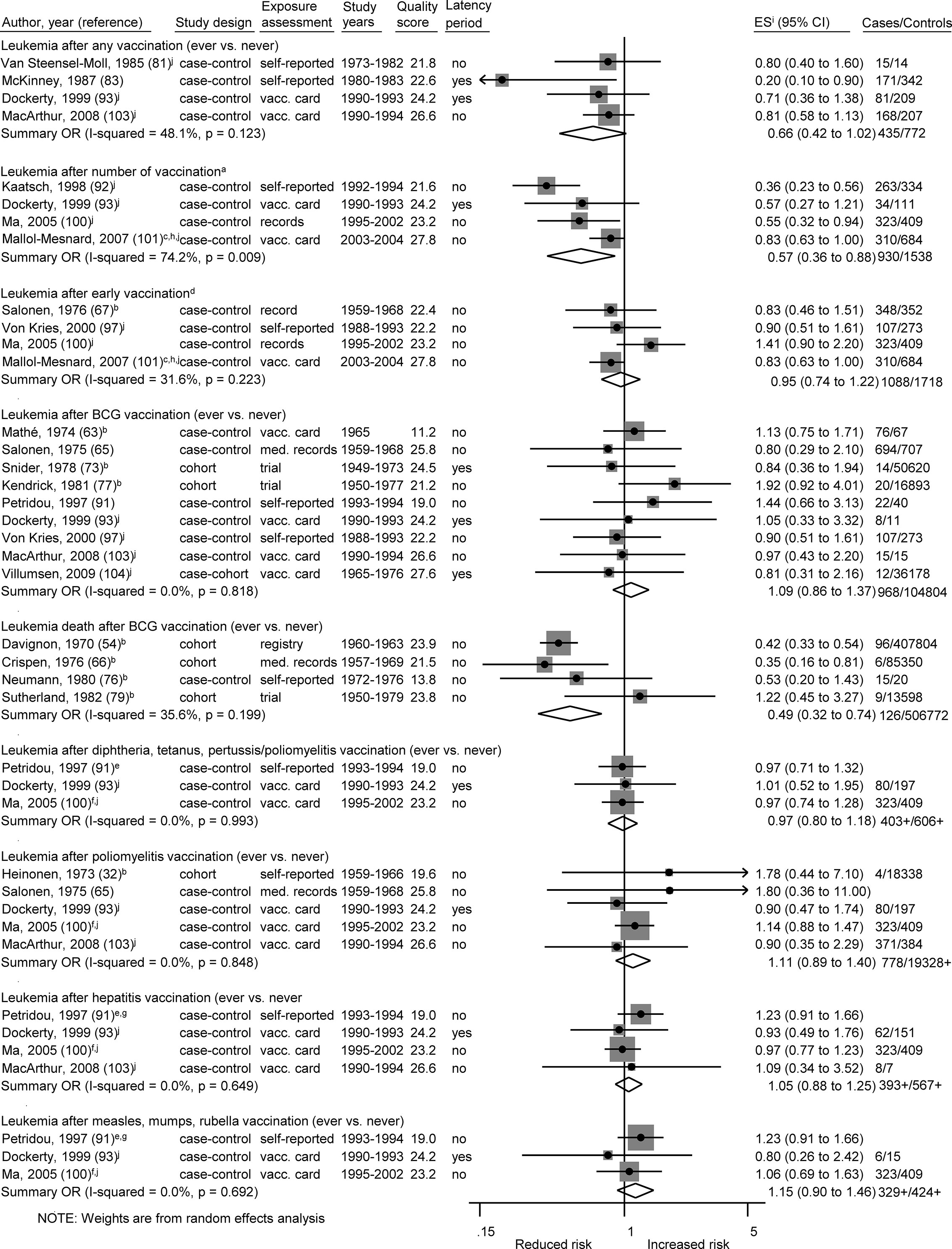
Figure 3 Vaccination and the risk of leukemia. BCG, Bacillus Calmette-Guérin; med. records, medical records; OR, odds ratio; ES, estimate. (a) Number of vaccinations: Kaatsch (any >6 vs. 0–3), Dockerty (any 3–4 vs. 0), Ma (Haemophilus influenzae type b 3+ vs. 1–2), Mallol-Messnard (any >3 vs. 3). (b) Calculation of crude ORs. (c) Estimates for acute leukemia. (d) Early vaccinations: Salonen (any ever vs. never, perinatal), Ma (hepatitis 3+ vs. 1–2, age <1), Mallol-Mesnard (any >3 vs. 3, age <0.5) von Kries (BCG vaccination, newborns). (e) Increment by ~3 doses. (f) Each additional dose. (g) Estimate for combination of measles, mumps, rubella, and hepatitis vaccination. (h) Inverted reference category. (i) ES includes single-study odds ratios or hazard ratios and summary odds ratios. (j) Adjusted estimate as indicated by published study.
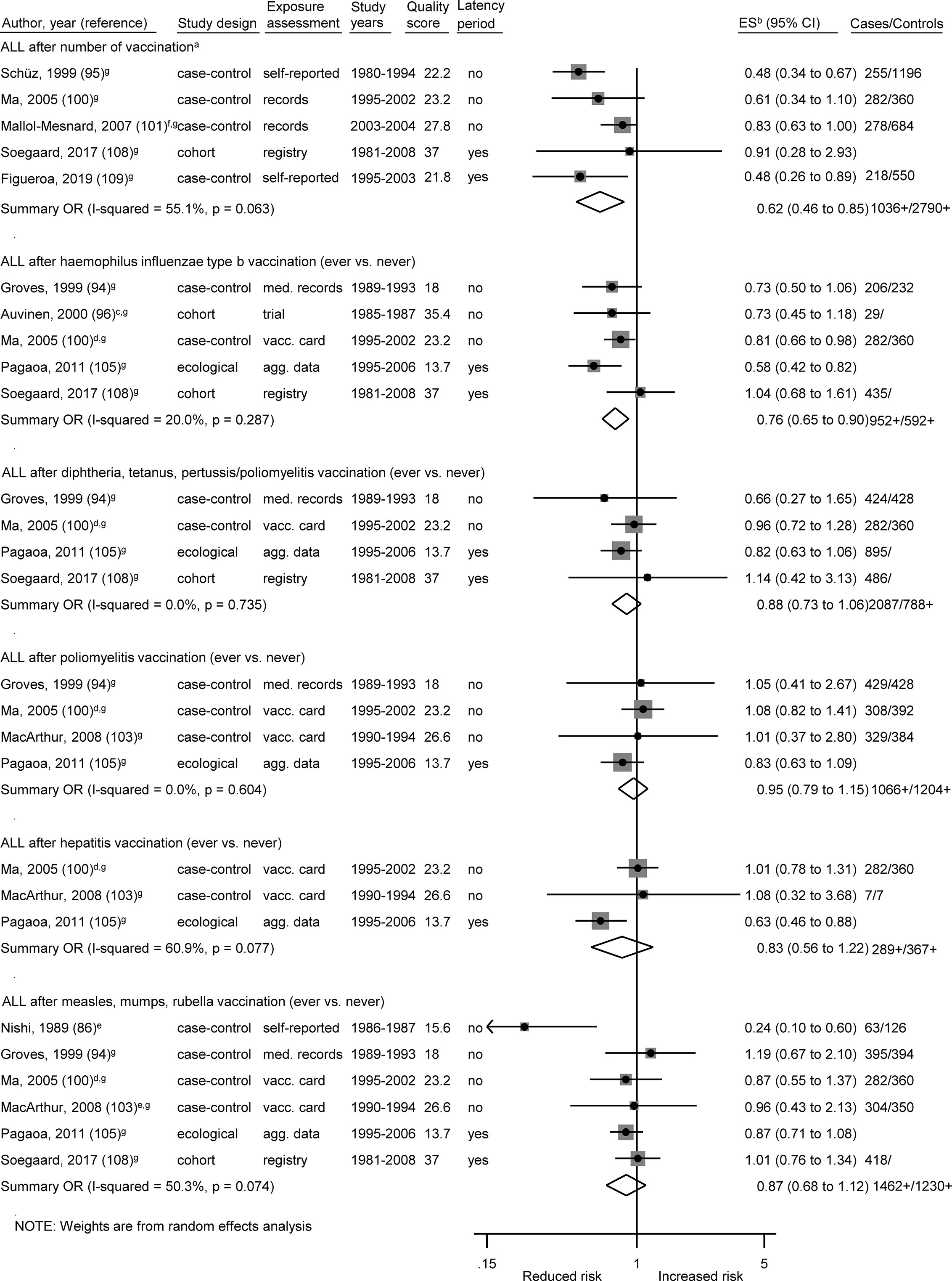
Figure 4 Vaccination and the risk of acute lymphoblastic leukemia (ALL). agg. data, aggregated data; ALL, acute lymphoblastic leukemia; med. records, medical records; OR, odds ratio; ES, estimate. (a) Number of vaccinations: Schüz (any >6 vs. 0–3), Ma (Haemophilus influenzae type b 3+ vs. 1–2), Mallol-Mesnard (any >3 vs. 3), Soegaard (complete vs. no/incomplete routine vaccination), Figueroa (complete vs. no/incomplete routine vaccination). (b) Calculation of crude ORs. (c) Early vs. late vaccination. (d) Each additional dose. (e) Estimate for measles. (f) Inverted reference category. (g) ES includes single-study odds ratios or hazard ratios and summary odds ratios. (h) Adjusted estimate as indicated by published study.
The summary OR of leukemia death was 0.49 (95% CI 0.32 to 0.74; I2 = 36%; N = 4; P value = 0.20) for bacillus Calmette–Guérin (BCG) vaccination compared to children without this vaccination (Figure 3). The four included studies were conducted between 1970 and 1982 and none of the studies accounted for a latency period. Three of the four studies (two cohort and one case-control study) reported a risk estimate below 1.0. Two obvious outlier studies were detected by omitting each study in turn from the meta-analysis (Table 5B). The observed risk reduction of the summary OR disappeared after the exclusion of Davignon et al. (54) or of Crispen et al. (66), which are two large and old cohort studies with valid exposure assessment via registry and medical documentation. Only the study of Neumann et al. (76) matched by age and sex, whereas the other three studies did not control for any confounders (54, 66, 79). Stratification by study period, study design, exposure assessment, and outcome assessment did not reveal any heterogeneity (I2 = 0%; Supplementary Figures 1A–D).
The association between ALL and Haemophilus influenzae type b (Hib) vaccination was assessed based on five studies, the OR was 0.76 (0.65 to 0.90; I² = 20%; N = 5, P = 0.29; Figure 4). Four of the five studies (one cohort, two case-control, one ecological study) reported a risk estimate below 1.0 (Figure 4). Omitting each study in turn from the meta-analysis did not reveal an obvious outlier among the five studies and all summary ORs still showed a risk reduction after any one study was excluded (Table 5C). The included studies were conducted between 1999 and 2017. All studies had a good assessment of the outcome and controlled for other vaccinations or other exposures in the immunological pathway e.g. infections. The stratification by study design, exposure assessment, inclusion of a latency period, and study quality did not show any heterogeneity (I2 = 0%; Supplementary Figures 1B, C, E, F).
Of two analyses focusing on the number of vaccine injections, one showed a risk reduction for leukemia (OR = 0.57; 0.36 to 0.88; N = 4; I2 = 74%; P value = 0.01) and one for ALL (OR = 0.62; 0.46 to 0.85; N = 5; I2 = 55%; P value = 0.06) after three or more vaccinations of any type. For both associations, all studies reported a risk estimate smaller than 1.0. Omitting each study in turn from the two analyses revealed obvious outlier studies for both associations. The heterogeneity across studies disappeared after exclusion of the German studies (92, 95) or the French study (101) and the summary OR was no longer significant after exclusion of the US study (100) (Tables 5B, C). The included studies covered together a study period of 29 years (1980 to 2008) for ALL and of 15 years (1990 to 2004) for leukemia. Most investigations were case-control studies (92, 93, 95, 100, 101, 109) and only one cohort study (108) was included in the meta-analysis for ALL. This Danish study was also the only study with exposure assessment based on a medical registry. The stratification by study design, exposure assessment, and consideration of a latency period did not show any heterogeneity (I2 = 0%; Supplementary Figures 1B, C, E). After stratification by study quality and adjustment, substantial heterogeneity was observed with a stronger risk reduction of ALL and leukemia for basic adjustment (ALL: OR = 0.48; 0.36 to 0.64; N = 2; leukemia: OR = 0.41; 0.27 to 0.61; N = 2) as compared to advanced adjustment (ALL: OR = 0.80; 0.65 to 0.99; N = 3; leukemia: OR = 0.73; 0.50 to 1.06; N = 2; Supplementary Figure 1G) and a significant risk reduction of ALL and leukemia for low quality below 24.7 points (ALL: OR = 0.50; 0.39 to 0.65; N = 3; leukemia: OR = 0.45; 0.33 to 0.91; N = 3) compared to a non-significant risk reduction for high quality equal or above 24.7 points (ALL: OR = 0.88; 0.66 to 1.04; N = 2; leukemia: OR = 0.83, 0.66 to 1.05; N = 1; Supplementary Figures 1F, H). In addition, a dose-response analysis was conducted to assess the risk of leukemia for an increasing number of vaccine injections (Supplementary Figure 2). The observed risk reduction was also observed in this analysis, even though not significant (OR = 0.94; 0.89 to 1.00; N = 2; I2 = 0%; P value = 0.54). However, trends required for the dose-response analysis could only be calculated for the studies of Kaatsch (92) and Dockerty (93). The other two studies had to be excluded due to an insufficient number of reported estimates (100) and significant deviations between the reported estimates of the different vaccine injections (101). For the same reasons, it was not possible to conduct a dose-response analysis for number of vaccine injections and ALL.
The remaining 22 specific analyses did not show any association between different types of vaccination (any, early, BCG, poliomyelitis, hepatitis, diphtheria-tetanus-pertussis/-poliomyelitis, measles-mumps-rubella) and overall childhood cancer risk, cancer death, or site-specific cancers (lymphoma, Hodgkin lymphoma, bone cancer, brain cancer, kidney cancer, skin cancer, leukemia, ALL; Figures 2–4). We observed substantial heterogeneity for cancer death after BCG vaccination (OR = 0.65; 0.34 to 1.22; N = 4; I2 = 82%; P value < 0.01), for cancer after poliomyelitis vaccination (OR = 1.18; 0.73 to 1.91; N = 3; I2 = 85%; P value <0.01), and for lymphoma after BCG vaccination (OR = 1.55; 0.34 to 7.13; N = 3; I2 = 77%; P value = 0.01). In each of these analyses, heterogeneity disappeared (I2 = 0%) after exclusion of one specific outlier study. However, the outlier studies differ in different analyses (Tables 5A–C). The stratified results are shown in Supplementary Figures 1A–H. Overall, no evidence of publication bias was seen in analyses including five or more studies either when using the funnel plot or when using the test by Egger et al. (48) (Supplementary Figures 3-8A–C).
Discussion
We observed an inverse association between BCG vaccination and leukemia death, between Hib vaccination and ALL, and between a high number of unspecified vaccinations and ALL or leukemia. The other 23 conducted analyses did show any associations. Despite the fact that we included a large number of publications over a long time period, the question of a possible risk reduction of childhood cancer after vaccination has not yet been finally clarified in our review and meta-analysis, since the exposure assessment of many studies has limited validity. This might be one of the reasons why published results are inconsistent. Some of these studies also have insufficient statistical power. In addition, most studies suffer from further methodological limitations, especially regarding the consideration of confounder control and latency periods. For most specific associations of interest, only few studies were available for pooling.
The risk reduction of ALL after Hib vaccination by 24% was fairly consistent across studies and by study characteristics. Only a recent Danish cohort study (108), that had the highest quality score (37.0), showed no association. However, we observed no heterogeneity after the stratification by study quality. A potential explanation for the link between Hib vaccination and ALL risk might be activation of the immune system early in life (21). ALL can frequently be traced back to a pre-leukemic clone carrying a prenatal genetic lesion (13, 110, 111). Postnatal acquired mutations then drive clonal evolution towards overt ALL. The protective role of vaccination in the development of ALL is based on the hypothesis that vaccines like Hib stimulate early formation of antibodies, prevent other infections, and modulate future responses to common childhood infections (23, 112). In line with this, mechanistic studies with mice that were repeatedly exposed to inflammatory stimuli, paralleling chronic infections in childhood, demonstrated that two enzymes, AID and RAG1-RAG2, drive clonal evolution of the most common subtype of ALL, B-cell precursor ALL (113). In addition, in vivo genetic studies connected inherited susceptibility to B-cell precursor ALL with postnatal infections by showing that B-cell precursor ALL was initiated in Pax5 heterozygous mice only when they were exposed to common pathogens (114). Moreover, among children in a large population-based birth cohort study, associations were observed between seven investigated serum immunoglobulin G titers and 10 exposures, either administered vaccines (e.g. BCG vaccination) or infections (115). These results indicate the existence of associations between immunogenic exposures and unrelated antibody titers, which may be responsible for non-specific effects of vaccinations on all-cause morbidity and mortality among children. Thus, early exposure to Hib vaccination may be responsible for the observed inverse association regarding ALL risk in our meta-analysis.
Our meta-analysis also showed a risk reduction for leukemia death after BCG vaccination in childhood, but not for the development of leukemia itself. The analyses on leukemia death and cancer death were limited to studies on relative risks or odds ratios of death among vaccinated and unvaccinated children. The study populations consisted of vaccination cohorts with vaccinated and unvaccinated children (60, 79), cohort studies calculating cancer mortality within the vaccinated and unvaccinated population (54, 66), or case-control studies with vaccinated and unvaccinated cancer deaths and healthy controls (76, 82). The observed risk reduction for leukemia death after BCG vaccination was mainly driven by two cohort studies (54, 66) with valid exposure and outcome assessment but without control for any confounders. We did not observe any heterogeneity between the four included studies in the stratified results.
In addition, studies comparing a high versus a low number of unspecified vaccinations observed an inverse association with ALL (95, 100, 101, 108, 109) and leukemia (92, 93, 100, 101). While this design of unspecified vaccinations mitigates some sources of confounding and bias, we noticed moderate heterogeneity across the study estimates of number of vaccinations. This heterogeneity disappeared after the exclusion of two large German case-control studies where both exposure assessment (interview of parents) and control of confounders (birth year, sex, socioeconomic status, and area) were suboptimal (92, 95). Assessment of the number of vaccinations was based on objective records in other studies but there were still differences (medical records, vaccination cards, medical claims data) that may affect the degree of misclassification (116). Claims data are assumed to have the highest validity regarding information on vaccination and were used in a large Danish cohort study (108). This high-quality study, which also carefully adjusted for confounders and considered latency periods, did not observed an association between number of vaccinations and ALL. In contrast, most other studies analyzing number of vaccinations (92, 95, 100, 101) and all studies that examined BCG vaccination (54, 66, 76, 79) did not take into account a latency period. However, this would result in non-differential misclassification and bias toward the null.
We did not find any other significant risk reduction of childhood cancer other than leukemia in our meta-analysis. With respect to poliomyelitis vaccination, the results of studies included in our meta-analysis were not consistent. Between 1955 and 1963, some poliomyelitis vaccines have been contaminated by simian virus 40 (34) that has the potential to initiate malignancy in various target tissues. This may explain the increased risk of childhood cancer for poliomyelitis vaccinations before 1963 with good exposure assessment. However in our stratified analyses, the increased risk of cancer after poliomyelitis immunization was only observed without consideration of a latency period, insufficient confounder control, low assessment of outcome, and low overall study quality. Moreover, there were also methodological limitations in the recent study that used an ecological design and did not show a correlation between childhood cancer and poliomyelitis vaccination (105). In such a study, an ecological bias may be introduced since only aggregated data are available. In addition, we observed substantial heterogeneity for the analysis of lymphoma after BCG vaccination. The recent Danish case-cohort study from Villumsen et al. (104) with very reliable vaccination information on an individual level, consideration of a latency period, and good confounder control indicated a beneficial effect of BCG vaccination on the risk of lymphomas. However, the other two old cohort studies in this analysis (73, 77), each with less than 10 cases and low overall quality, did not support this result.
In contrast to the conducted meta-analysis by Morra et al. (32), we did not observed an inverse association between leukemia and early vaccination before the age of 1 year in our synthesis. These different meta-analysis results can be explained by our additional inclusion of the study from Ma et al. (100) from 2005, which did not show any association between early vaccination and leukemia with careful adjustment. In addition contrary to our analysis, the meta-analysis of Morra et al. (32) included two old and large studies (54, 66) on leukemia death after early BCG vaccination, which found a strong risk reduction without control for any confounders. We preferred to analyze these studies on BCG vaccination and early immunization based on the different outcomes, leukemia death, and leukemia incidence, separately.
Our meta-analysis has specific strengths including the extensive search strategy we used to ensure that all relevant publications on this topic were identified. This enabled us to conduct separate analyses on histological and site-specific childhood cancers as well as on certain vaccines, age at vaccination, and number of vaccinations. To consider the overall study quality, we used the established NOS as well as our own more detailed quality assessment scale. The latter additionally considered important issues such as latency periods, quality of statistical methods, training of interviewers, exposure assessment in cohort studies, and cancer among controls of case-control studies. There was no conclusive evidence of publication bias, but the power of the test is poor in a meta-analysis with only a few included studies. However, a graphical examination of the plots also did not suggest a publication bias.
The small number of studies for each exposure-disease association and the relatively high level of heterogeneity across studies in some of our analyses is the main limitation of this meta-analysis. When the number of studies and the true fraction of heterogeneity is small, there appears a substantial positive bias for I2 but this bias is typically negative when the true fraction of heterogeneity is large and the number of studies is small (117). To account for potential heterogeneity we used random effects models and to assess its effect on our results, we stratified our analyses by study characteristics.
In conclusion, we found evidence of an inverse association between BCG vaccination and leukemia death, Hib vaccination and ALL, and a high number of unspecified vaccinations and ALL as well as leukemia. However, these results should be interpreted with caution given the small number of studies, no consideration of latency, and limited exposure assessment in some studies as well as insufficient confounder adjustment, in particular for infections. All studies included in this review and meta-analysis had at least one of these substantial limitations. Finally, although risk reductions after vaccination appear biologically plausible in leukemia, studies on dose effect and on age at vaccination with good exposure assessment and advanced confounder controlling are rare. Large cohort studies with valid assessment of immunizations, adequate consideration of the latency period, and detailed information on possible confounders (e.g. infections and other vaccines) are needed to assess the association between different types of vaccinations and specific childhood cancers.
Data Availability Statement
The original contributions presented in the study are included in the article/Supplementary Material. Further inquiries can be directed to the corresponding author.
Author Contributions
MM designed the meta-analysis, the analytic strategies and the detailed quality score, and directed its implementation, including quality assurance and control. She was in charge of creating the manuscript and conducting the literature review and the meta-analysis. LB and PK assisted in conducting the literature review and meta-analysis including acquisition of data, quality assessment, and preparation of figures and tables. LC assisted conducting the literature review including database searches, selections, and assessment of publications. IL, UH, and WA contributed to the analyses and interpretation of the results. WA assumes an overall scientific responsibility during the creation of the publication and thus assumes a particular responsibility for the quality and integrity of the publication. All authors revised the manuscript critically for important intellectual content and agreed to be accountable for all aspects of the work in ensuring that questions related to the accuracy or integrity of any part of the work are appropriately investigated and resolved. All authors contributed to the article and approved the submitted version.
Conflict of Interest
The authors declare that the research was conducted in the absence of any commercial or financial relationships that could be construed as a potential conflict of interest.
Acknowledgments
The authors thank the former study group member Patricia Sadre Fischer as well as Claas Sonntag for their contributions to the literature search.
Supplementary Material
The Supplementary Material for this article can be found online at: https://www.frontiersin.org/articles/10.3389/fonc.2020.610843/full#supplementary-material
Abbreviations
Adj, adjustment; AL, acute leukemia; ALL, acute lymphoblastic leukemia; AML, acute myeloid leukemia; BCG, bacillus Calmette–Guérin (vole bacillus; tuberculosis); BCP, B-cell precursor; cALL, common acute lymphoblastic leukemia; Chi, chicken pox (varicella zoster); Cho, cholera; CI, confidence interval; CNS, central nervous system; D, diphtheria; DT, diphtheria-tetanus; DTP, diphtheria-tetanus-pertussis/whooping cough; DTPolio, diphtheria-tetanus-poliomyelitis; Exc, exclusion for meta-analysis; Hep, hepatitis; Hib, Haemophilus influenzae type b; Inf, influenza; IPV, inactivated poliomyelitis vaccine; HL, Hodgkin lymphoma; m, months; Mat, matching; Mea, measles; Men, meningococcus; MMR, measles-mumps-rubella; MRC, Medical Research Council; Mum, mumps; NHL, non-Hodgkin lymphoma; OR, odds ratio; P, points; Pne, pneumococcus; Polio, poliomyelitis; SE, standard error; Sma, smallpox; Typ, typhoid; y, years; Yel, yellow fever.
References
1. Adami H-O, Hunter D, Trichopoulos D. Textbook of Cancer Epidemiology. 2nd ed. Oxford: Oxford University Press (2008). doi: 10.1093/acprof:oso/9780195311174.001.0001
2. Morton LM, Savage SA, Bhatia S. Multiple primary cancers Morton LM, Savage SA, Bhatia S. In: Thun M, Linet MS, Cerhan JR, Haiman CA, Schottenfeld D, editors. Cancer Epidemiology and Prevention. Oxford: Oxford University Press (2017). doi: 10.1093/oso/9780190238667.003.0060
3. Schottenfeld D, Fraumeni JF. Cancer Epidemiology and Prevention. 3rd ed. Oxford: Oxford University Press (2006). doi: 10.1093/acprof:oso/9780195149616.001.0001
4. Narod SA. Genetic epidemiology of childhood cancer. Biochim Biophys Acta (1996) 1288(3):F141–50. doi: 10.1016/S0304-419X(96)00031-5
5. Spector LG, Street D, Pankratz N, Marcotte EL. Genetic and nongenetic risk factors for childhood cancer. Pediatr Clin North Am (2015) 62(1):11–25. doi: 10.1016/j.pcl.2014.09.013
6. Chokkalingam AP, Buffler PA. Genetic susceptibility to childhood leukaemia. Radiat Prot Dosimetry (2008) 132(2):119–29. doi: 10.1093/rpd/ncn255
7. Mullighan CG, Goorha S, Radtke I, Miller CB, Coustan-Smith E, Dalton JD, et al. Genome-wide analysis of genetic alterations in acute lymphoblastic leukaemia. Nature (2007) 446(7137):758–64. doi: 10.1038/nature05690
8. Mullighan CG, Phillips LA, Su X, Ma J, Miller CB, Shurtleff SA, et al. Genomic analysis of the clonal origins of relapsed acute lymphoblastic leukemia. Science (2008) 322(5906):1377–80. doi: 10.1126/science.1164266
9. Sherborne AL, Hosking FJ, Prasad RB, Kumar R, Koehler R, Vijayakrishnan J, et al. Variation in CDKN2A at 9p21.3 influences childhood acute lymphoblastic leukemia risk. Nat Genet (2010) 42(6):492–4. doi: 10.1038/ng.585
10. Treviño LR, Yang W, French D, Hunger SP, Carroll WL, Devidas M, et al. Germline genomic variants associated with childhood acute lymphoblastic leukemia. Nat Genet (2009) 41(9):1001–5. doi: 10.1038/ng.432
11. Zhang J, Walsh MF, Wu G, Edmonson MN, Gruber TA, Easton J, et al. Germline mutations in predisposition genes in pediatric cancer. N Engl J Med (2015) 373(24):2336–46. doi: 10.1056/NEJMoa1508054
12. Carlos-Wallace FM, Zhang L, Smith MT, Rader G, Steinmaus C. Parental, in utero, and early-life exposure to benzene and the risk of childhood leukemia: a meta-analysis. Am J Epidemiol (2016) 183(1):1–14. doi: 10.1093/aje/kwv120
13. Greaves M. Infection, immune responses and the aetiology of childhood leukaemia. Nat Rev Cancer (2006) 6(3):193–203. doi: 10.1038/nrc1816
14. Cooke JV. The incidence of acute leukemia in children. JAMA (1942) 119(7):547–. doi: 10.1001/jama.1942.02830240011004
15. Kellett CE. Acute myeloid leukaemia in one of identical twins. Arch Dis Child (1937) 12(70):239–52. doi: 10.1136/adc.12.70.239
17. Poynton F, Thursfield H, Paterson D. The Severe Blood Diseases of Childhood. Br J Child Dis (1922) XIX:128–44. doi: 10.1097/00000441-192210000-00050
18. Kinlen L. Evidence for an infective cause of childhood leukaemia: comparison of a Scottish new town with nuclear reprocessing sites in Britain. Lancet (1988) 2(8624):1323–7. doi: 10.1016/S0140-6736(88)90867-7
19. Kinlen LJ. Epidemiological evidence for an infective basis in childhood leukaemia. Br J Cancer (1995) 71(1):1–5. doi: 10.1038/bjc.1995.1
20. Kinlen LJ, Dickson M, Stiller CA. Childhood leukaemia and non-Hodgkin’s lymphoma near large rural construction sites, with a comparison with Sellafield nuclear site. BMJ (1995) 310(6982):763–8. doi: 10.1136/bmj.310.6982.763
21. Greaves MF. Speculations on the cause of childhood acute lymphoblastic leukemia. Leukemia (1988) 2(2):120–5.
22. Lakshmi Narendra B, Eshvendar Reddy K, Shantikumar S, Ramakrishna S. Immune system: a double-edged sword in cancer. Inflamm Res (2013) 62(9):823–34. doi: 10.1007/s00011-013-0645-9
23. Flores-Lujano J. Infection During the First Year of Life and Acute Leukemia: Epidemiological Evidence. In: Clinical Epidemiology of Acute Lymphoblastic Leukemia - From the Molecules to the Clinic. Intech (2013). doi: 10.5772/52717
24. Rousseau MC, Parent ME, St-Pierre Y. Potential health effects from non-specific stimulation of the immune function in early age: the example of BCG vaccination. Pediatr Allergy Immunol (2008) 19(5):438–48. doi: 10.1111/j.1399-3038.2007.00669.x
25. Benn CS, Netea MG, Selin LK, Aaby P. A small jab - a big effect: nonspecific immunomodulation by vaccines. Trends Immunol (2013) 34(9):431–9. doi: 10.1016/j.it.2013.04.004
26. Maia R, Wunsch Filho V. Infection and childhood leukemia: review of evidence. Rev Saude Publica (2013) 47(6):1172–85. doi: 10.1590/S0034-89102013000901172
27. Marcotte EL, Thomopoulos TP, Infante-Rivard C, Clavel J, Petridou ET, Schuz J, et al. Caesarean delivery and risk of childhood leukaemia: a pooled analysis from the Childhood Leukemia International Consortium (CLIC). Lancet Haematol (2016) 3(4):e176–85. doi: 10.1016/S2352-3026(16)00002-8
28. Rudant J, Lightfoot T, Urayama KY, Petridou E, Dockerty JD, Magnani C, et al. Systematic reviews and meta-and pooled analyses childhood acute lymphoblastic leukemia and indicators of early immune stimulation: a childhood leukemia international consortium study. Am J Epidemiol (2014) 181(8):549–62. doi: 10.1093/aje/kwu298
29. Rudant J, Orsi L, Menegaux F, Petit A, Baruchel A, Bertrand Y, et al. Childhood acute leukemia, early common infections, and allergy: the ESCALE study. Am J Epidemiol (2010) 172(9):1015–27. doi: 10.1093/aje/kwq233
30. U. K. Childhood Cancer Study Investigators. Breastfeeding and childhood cancer. Br J Cancer (2001) 85(11):1685–94. doi: 10.1054/bjoc.2001.2110
31. Urayama KY, Buffler PA, Gallagher ER, Ayoob JM, Ma X. A meta-analysis of the association between day-care attendance and childhood acute lymphoblastic leukaemia. Int J Epidemiol (2010) 39(3):718–32. doi: 10.1093/ije/dyp378
32. Morra ME, Kien ND, Elmaraezy A, Abdelaziz OAM, Elsayed AL, Halhouli O, et al. Early vaccination protects against childhood leukemia: a systematic review and meta-analysis. Sci Rep (2017) 7(1):15986. doi: 10.1038/s41598-017-16067-0
33. Engels EA, Chen J, Viscidi RP, Shah KV, Daniel RW, Chatterjee N, et al. Poliovirus vaccination during pregnancy, maternal seroconversion to simian virus 40, and risk of childhood cancer. Am J Epidemiol (2004) 160(4):306–16. doi: 10.1093/aje/kwh219
34. Dang-Tan T, Mahmud SM, Puntoni R, Franco EL. Polio vaccines, simian virus 40, and human cancer: the epidemiologic evidence for a causal association. Oncogene (2004) 23(38):6535–40. doi: 10.1038/sj.onc.1207877
35. Stroup DF, Berlin JA, Morton SC, Olkin I, Williamson GD, Rennie D, et al. Meta-analysis of observational studies in epidemiology: a proposal for reporting. Meta-analysis Of Observational Studies in Epidemiology (MOOSE) group. JAMA (2000) 283(15):2008–12. doi: 10.1001/jama.283.15.2008
36. Moher D, Liberati A, Tetzlaff J, Altman DG, Group P. Preferred reporting items for systematic reviews and meta-analyses: the PRISMA statement. BMJ (2009) 339:b2535. doi: 10.1136/bmj.b2535
37. Engels EA, Katki HA, Nielsen NM, Winther JF, Hjalgrim H, Gjerris F, et al. Cancer incidence in Denmark following exposure to poliovirus vaccine contaminated with simian virus 40. J Natl Cancer Inst (2003) 95(7):532–9. doi: 10.1093/jnci/95.7.532
38. Strickler HD, Rosenberg PS, Devesa SS, Fraumeni JF Jr., Goedert JJ. Contamination of poliovirus vaccine with SV40 and the incidence of medulloblastoma. Med Pediatr Oncol (1999) 32(1):77–8. doi: 10.1002/(SICI)1096-911X(199901)32:1<77::AID-MPO21>3.0.CO;2-F
39. Strickler HD, Rosenberg PS, Devesa SS, Hertel J, Fraumeni JF Jr, Goedert JJ. Contamination of poliovirus vaccines with simian virus 40 (1955-1963) and subsequent cancer rates. JAMA (1998) 279(4):292–5. doi: 10.1001/jama.279.4.292
40. Wells G, Shea B, O’Connell D, Peterson j, Welch V, Losos M, et al. The Newcastle-Ottawa Scale (NOS) for assessing the quality of nonrandomised studies in meta-analyses Ottawa. Ontario, Canada: Ottawa Health Research Institute (2000). Available at: http://www.ohri.ca/programs/clinical_epidemiology/oxford.asp.
41. Higgins JPT, Green S. Cochrane handbook for systematic reviews of interventions: The Cochrane Collaboration. (2011). Available at: http://handbook-5-1.cochrane.org/.
42. Stang A. Critical evaluation of the Newcastle-Ottawa scale for the assessment of the quality of nonrandomized studies in meta-analyses. Eur J Epidemiol (2010) 25(9):603–5. doi: 10.1007/s10654-010-9491-z
43. Sanderson S, Tatt ID, Higgins JP. Tools for assessing quality and susceptibility to bias in observational studies in epidemiology: a systematic review and annotated bibliography. Int J Epidemiol (2007) 36(3):666–76. doi: 10.1093/ije/dym018
44. Deeks JJ, Altman DG, Bradburn MJ. Statistical methods for examining heterogeneity and combining results from several studies in meta-analysis. In: Egger M, Davey Smith G, Altman D, editors. Systematic Reviews in Health Care: Meta-Analysis in Context, 2nd ed. London: BMJ Publishing Group (2001). p. 285–312. doi: 10.1002/9780470693926.ch15
45. Greenland S, Longnecker MP. Methods for trend estimation from summarized dose-response data, with applications to meta-analysis. Am J Epidemiol (1992) 135(11):1301–9. doi: 10.1093/oxfordjournals.aje.a116237
46. Higgins JPT, Thompson SG, Deeks JJ, Altman DG. Measuring inconsistency in meta-analyses. BMJ (2003) 327(7414):557–60. doi: 10.1136/bmj.327.7414.557
47. Ziegler A, Lange S, Bender R. Systematic reviews and meta-analyses. DMW - Dtsche Med Wochenschr (2004) 129(19):T11–T5. doi: 10.1055/s-2004-836077
48. Egger M, Smith GD, Schneider M, Minder C. Bias in meta-analysis detected by a simple, graphical test. BMJ (1997) 315(7109):629–34. doi: 10.1136/bmj.315.7109.629
49. Harris RJ, Bradburn MJ, Deeks JJ, Harbord RM, Altman DG, Sterne JAC. Metan fixed- and random-effects meta-analysis. Stata J (2008) 8(1):3–28. doi: 10.1177/1536867X0800800102
50. Fraumeni JF Jr., Ederer F, Miller RW. An evaluation of the carcinogenicity of simian virus 40 in man. JAMA (1963) 185:713–8. doi: 10.1001/jama.1963.03060090045016
51. Innis MD. Immunisation and childhood leukaemia. Lancet (1965) 1(7385):605–. doi: 10.1016/S0140-6736(65)91188-8
52. Stewart AM, Hewitt D. Aetiology of childhood leukaemia. Lancet (1965) 2(7416):789–90. doi: 10.1016/S0140-6736(65)90835-4
53. Innis MD. Oncogenesis and poliomyelitis vaccine. Nature (1968) 219(5157):972–3. doi: 10.1038/219972a0
54. Davignon L, Robillard P, Lemonde P, Frappier ABCG. Vaccination and leukæmia mortality. Lancet (1970) 296(7674):638–. doi: 10.1016/S0140-6736(70)91402-9
55. Waaler HT. B.C.G. and leukaemia mortality. Lancet (1970) 2(7686):1314–. doi: 10.1016/S0140-6736(70)92276-2
57. Comstock GW. Leukaemia and B.C.G. A controlled trial. Lancet (1971) 2(7733):1062–3. doi: 10.1016/S0140-6736(71)90381-3
58. Hems G, Stuart A. B.C.G. and leukaemia. Lancet (1971) 1(7691):183–. doi: 10.1016/S0140-6736(71)91949-0
59. Kinlen LJ, Pike MC. B.C.G. vaccination and leukaemia. Evidence of vital statistics. Lancet (London England) (1971) 2(7721):398–402. doi: 10.1016/S0140-6736(71)90114-0
60. Medical Research C. BCG and vole bacillus vaccines in the prevention of tuberculosis in adolescence and early adult life. Bull World Health Organ (1972) 46(3):371–85.
61. Rosenthal SR, Crispen RG, Thorne MG, Piekarski N, Raisys N, Rettig PG. BCG vaccination and leukemia mortality. JAMA (1972) 222(12):1543–4. doi: 10.1001/jama.222.12.1543
62. Heinonen OP, Shapiro S, Monson RR, Hartz SC, Rosenberg L, Slone D. Immunization during pregnancy against poliomyelitis and influenza in relation to childhood malignancy. Int J Epidemiol (1973) 2(3):229–35. doi: 10.1093/ije/2.3.229
63. Mathé G, Facy F, Hatton F, Halle-Pannenko O. BCG vaccination and acute leukaemia. Biomedicine (1974) 21(3):132–4.
64. Comstock GW, Martinez I, Livesay VT. Efficacy of BCG vaccination in prevention of cancer. J Natl Cancer Inst (1975) 54(4):835–9.
65. Salonen T, Saxen L. Risk indicators in childhood malignancies. Int J Cancer (1975) 15(6):941–6. doi: 10.1002/ijc.2910150609
66. Crispen RG, Rosenthal SR. BCG vaccination and cancer mortality. Cancer Immunol Immunother (1976) 1(3):139–42. doi: 10.1007/BF00205456
69. Ambrosch F, Krepler P, Wiedermann G. The effect of neonatal BCG vaccination on the incidence of leukemia. MMW Munch Med Wochenschr (1978) 120(8):243–6.
70. Andersen E, Isager H. Pre-morbid factors in Hodgkin’s disease. II. BCG-vaccination status, tuberculosis, infectious diseases, tonsillectomy, and appendectomy. Scand J Haematol (1978) 21(4):273–7. doi: 10.1111/j.1600-0609.1978.tb00363.x
71. Sinniah D, White JC, Omar A, Chua CP. Acute leukemia in Malaysian children. Cancer (1978) 42(4):1970–5. doi: 10.1002/1097-0142(197810)42:4<1970::AID-CNCR2820420441>3.0.CO;2-7
72. Skegg DC. BCG vaccination and the incidence of lymphomas and leukaemia. Int J Cancer (1978) 21(1):18–21. doi: 10.1002/ijc.2910210105
73. Snider DE, Comstock GW, Martinez I, Caras GJ. Efficacy of BCG vaccination in prevention of cancer: an update. J Natl Cancer Inst (1978) 60(4):785–8. doi: 10.1093/jnci/60.4.785
74. Farwell JR, Dohrmann GJ, Marrett LD, Meigs JW. Effect of SV40 virus-contaminated polio vaccine on the incidence and type of CNS neoplasms in children: a population-based study. Trans Am Neurol Assoc (1979) 104:261–4.
75. Nilsson BS, Widström O. Neonatal B.C.G. vaccination and childhood cancer. Lancet (1979) 1(8109):222–. doi: 10.1016/S0140-6736(79)90632-9
77. Kendrick MA, Comstock GW. BCG vaccination and the subsequent development of cancer in humans. J Natl Cancer Inst (1981) 66(3):431–7.
78. Grufferman S, Wang HH, DeLong ER, Kimm SY, Delzell ES, Falletta JM. Environmental factors in the etiology of rhabdomyosarcoma in childhood. J Natl Cancer Inst (1982) 68(1):107–13.
79. Sutherland I. BCG and vole bacillus vaccination in adolescence and mortality from leukaemia. Stat Med (1982) 1(4):329–35. doi: 10.1002/sim.4780010410
80. Farwell JR, Dohrmann GJ, Flannery JT. Medulloblastoma in childhood: an epidemiological study. J Neurosurg (1984) 61(4):657–64. doi: 10.3171/jns.1984.61.4.0657
81. van Steensel-Moll HA, Valkenburg HA, Vandenbroucke JP, van Zanen GE. Are maternal fertility problems related to childhood leukaemia? Int J Epidemiol (1985) 14(4):555–9. doi: 10.1093/ije/14.4.555
82. Kneale GW, Stewart AM, Kinnier Wilson LM. Immunizations against infectious diseases and childhood cancers. Cancer Immunol Immunother (1986) 21(2):129–32. doi: 10.1007/BF00199860
83. McKinney PA, Cartwright RA, Saiu JM, Mann JR, Stiller CA, Draper GJ, et al. The inter-regional epidemiological study of childhood cancer (IRESCC): a case control study of aetiological factors in leukaemia and lymphoma. Arch Dis Child (1987) 62(3):279–87. doi: 10.1136/adc.62.3.279
84. Hartley AL, Birch JM, McKinney PA, Blair V, Teare MD, Carrette J, et al. The inter-regional epidemiological study of childhood cancer (IRESCC): past medical history in children with cancer. J Epidemiol Community Health (1988) 42:235–42. doi: 10.1136/jech.42.3.235
85. Gilman EA, Margaret L, Wilson K, Kneale GW, Waterhouse JAH. Childhood cancers and their association with pregnancy drugs and illnesses. Paediatr Perinat Epidemiol (1989) 3(1):66–94. doi: 10.1111/j.1365-3016.1989.tb00371.x
86. Nishi M, Miyake H. A case-control study of non-T cell acute lymphoblastic leukaemia of children in Hokkaido, Japan. J Epidemiol Community Health (1989) 43(4):352–5. doi: 10.1136/jech.43.4.352
87. Buckley JD, Buckley CM, Ruccione K, Sather HN, Waskerwitz MJ, Woods WG, et al. Epidemiological characteristics of childhood acute lymphocytic leukemia. Analysis by immunophenotype. The Childrens Cancer Group. Leukemia (1994) 8(5):856–64.
88. Shu XO, Nesbit ME, Buckley JD, Krailo MD, Robinson LL. An exploratory analysis of risk factors for childhood malignant germ-cell tumors: report from the Childrens Cancer Group (Canada, United States). Cancer Causes Control (1995) 6(3):187–98. doi: 10.1007/BF00051790
89. Kaatsch P, Kaletsch U, Krummenauer F, Meinert R, Miesner A, Haaf G, et al. Case control study on childhood leukemia in Lower Saxony, Germany. Basic considerations, methodology, and summary of results. Klin Padiatr (1996) 208(4):179–85. doi: 10.1055/s-2008-1046470
90. Bhatia S, Nesbit ME, Egeler RM, Buckley JD, Mertens A, Robison LL. Epidemiologic study of Langerhans cell histiocytosis in children. J Pediatr (1997) 130(5):774–84. doi: 10.1016/S0022-3476(97)80021-2
91. Petridou E, Trichopoulos D, Kalapothaki V, Pourtsidis A, Kogevinas M, Kalmanti M, et al. The risk profile of childhood leukaemia in Greece: a nationwide case-control study. Br J Cancer (1997) 76(9):1241–7. doi: 10.1038/bjc.1997.541
92. Kaatsch P, Kaletsch U, Meinert R, Miesner A, Hoisl M, Schuz J, et al. German case control study on childhood leukaemia–basic considerations, methodology and summary of the results. Klin Padiatr (1998) 210(4):185–91. doi: 10.1055/s-2008-1043877
93. Dockerty JD, Skegg DC, Elwood JM, Herbison GP, Becroft DM, Lewis ME. Infections, vaccinations, and the risk of childhood leukaemia. Br J Cancer (1999) 80(9):1483–9. doi: 10.1038/sj.bjc.6690548
94. Groves FD, Gridley G, Wacholder S, Shu XO, Robison LL, Neglia JP, et al. Infant vaccinations and risk of childhood acute lymphoblastic leukaemia in the USA. Br J Cancer (1999) 81(1):175–8. doi: 10.1038/sj.bjc.6690668
95. Schüz J, Kaletsch U, Meinert R, Kaatsch P, Michaelis J. Association of childhood leukaemia with factors related to the immune system. Br J Cancer (1999) 80(3-4):585–90. doi: 10.1038/sj.bjc.6690395
96. Auvinen A, Hakulinen T, Groves F. Haemophilus influenzae type B vaccination and risk of childhood leukaemia in a vaccine trial in Finland. Br J Cancer (2000) 83(7):956–8. doi: 10.1054/bjoc.2000.1382
97. Von Kries R, Peter Grunert V, Kaletsch U, Michaelis J, Göbel U, Grunert VP, et al. Prevention of childhood leukemia by BCG vaccination in newborns? A population-based case-control study in Lower Saxony, Germany. Pediatr Hematol Oncol (2000) 17(7):541–50. doi: 10.1080/08880010050122807
98. Krone B, Kolmel KF, Grange JM, Mastrangelo G, Henz BM, Botev IN, et al. Impact of vaccinations and infectious diseases on the risk of melanoma–evaluation of an EORTC case-control study. Eur J Cancer (2003) 39(16):2372–8. doi: 10.1016/S0959-8049(03)00625-7
99. Frentzel-Beyme R, Becher H, Salzer-Kuntschik M, Kotz R, Salzer M. Factors affecting the incident juvenile bone tumors in an Austrian case-control study. Cancer Detect Prev (2004) 28(3):159–69. doi: 10.1016/j.cdp.2004.03.001
100. Ma X, Does MB, Metayer C, Russo C, Wong A, Buffler PA. Vaccination history and risk of childhood leukaemia. Int J Epidemiol (2005) 34(5):1100–9. doi: 10.1093/ije/dyi113
101. Mallol-Mesnard N, Menegaux F, Auvrignon A, Auclerc MF, Bertrand Y, Nelken B, et al. Vaccination and the risk of childhood acute leukaemia: the ESCALE study (SFCE). Int J Epidemiol (2007) 36(1):110–6. doi: 10.1093/ije/dyl270
102. Daniels JL, Pan IJ, Olshan AF, Breslow NE, Bunin GR, Ross JA. Obstetric history and birth characteristics and Wilms tumor: a report from the Children’s Oncology Group. Cancer Causes Control (2008) 19(10):1103–10. doi: 10.1007/s10552-008-9174-1
103. MacArthur AC, McBride ML, Spinelli JJ, Tamaro S, Gallagher RP, Theriault GP. Risk of childhood leukemia associated with vaccination, infection, and medication use in childhood. Am J Epidemiol (2008) 167(5):598–606. doi: 10.1093/aje/kwm339
104. Villumsen M, Sørup S, Jess T, Ravn H, Relander T, Baker JL, et al. Risk of lymphoma and leukaemia after bacille Calmette-Guérin and smallpox vaccination: A Danish case-cohort study. Vaccine (2009) 27(49):6950–8. doi: 10.1016/j.vaccine.2009.08.103
105. Pagaoa MA, Okcu MF, Bondy ML, Scheurer ME. Associations between vaccination and childhood cancers in Texas regions. J Pediatr (2011) 158(6):996–1002. doi: 10.1016/j.jpeds.2010.11.054
106. Qu CF, Chen TY, Fan CS, Zhan QM, Wang YT, Lu JH, et al. Efficacy of neonatal HBV vaccination on liver cancer and other liver diseases over 30-year follow-up of the qidong hepatitis B intervention study: a cluster randomized controlled trial. PloS Med (2014) 11(12):13. doi: 10.1371/journal.pmed.1001774
107. Sankaran H, Danysh HE, Scheurer ME, Okcu MF, Skapek SX, Hawkins DS, et al. The role of childhood infections and immunizations on childhood rhabdomyosarcoma: a report from the children’s oncology group. Pediatr Blood Cancer (2016) 63(9):1557–62. doi: 10.1002/pbc.26065
108. Soegaard SH, Rostgaard K, Schmiegelow K, Kamper-Jorgensen M, Hargreave M, Hjalgrim H, et al. Childhood vaccinations and risk of acute lymphoblastic leukaemia in children. Int J Epidemiol (2017) 46(3):905–13. doi: 10.1093/ije/dyx052
109. Figueroa SC, Kennedy CJ, Wesseling C, Wiemels JM, Morimoto L, Mora AM. Early immune stimulation and childhood acute lymphoblastic leukemia in Costa Rica: A comparison of statistical approaches. Environ Res (2020) 182:109023. doi: 10.1016/j.envres.2019.109023
110. Wiemels JL, Cazzaniga G, Daniotti M, Eden OB, Addison GM, Masera G, et al. Prenatal origin of acute lymphoblastic leukaemia in children. Lancet (1999) 354(9189):1499–503. doi: 10.1016/S0140-6736(99)09403-9
111. Greaves M. In utero origins of childhood leukaemia. Early Hum Dev (2005) 81(1):123–9. doi: 10.1016/j.earlhumdev.2004.10.004
112. Wiemels J. Perspectives on the causes of childhood leukemia. Chem Biol Interact (2012) 196:59–67. doi: 10.1016/j.cbi.2012.01.007
113. Swaminathan S, Klemm L, Park E, Papaemmanuil E, Ford A, Kweon SM, et al. Mechanisms of clonal evolution in childhood acute lymphoblastic leukemia. Nat Immunol (2015) 16(7):766–74. doi: 10.1038/ni.3160
114. Martin-Lorenzo A, Hauer J, Vicente-Duenas C, Auer F, Gonzalez-Herrero I, Garcia-Ramirez I, et al. Infection exposure is a causal factor in b-cell precursor acute lymphoblastic leukemia as a result of pax5-inherited susceptibility. Cancer Discov (2015) 5(12):1328–43. doi: 10.1158/2159-8290.CD-15-0892
115. Caputo M, Raupach-Rosin H, Karch A, Borte M, Lehmann I, Liebert UG, et al. Vaccinations and infections are associated with unrelated antibody titers: an analysis from the german birth cohort study LISA. Front Pediatr (2019) 7:1–11. doi: 10.3389/fped.2019.00254
116. Seidman DS, Slater PE. Accuracy of the medical interview. Br J Obstet Gynaecol (1987) 94(8):721–3. doi: 10.1111/j.1471-0528.1987.tb03715.x
Keywords: acute lymphoblastic leukemia, childhood leukemia, leukemia death, immunization, immune system
Citation: Marron M, Brackmann LK, Kuhse P, Christianson L, Langner I, Haug U and Ahrens W (2021) Vaccination and the Risk of Childhood Cancer—A Systematic Review and Meta-Analysis. Front. Oncol. 10:610843. doi: 10.3389/fonc.2020.610843
Received: 27 September 2020; Accepted: 07 December 2020;
Published: 22 January 2021.
Edited by:
Yawei Zhang, Yale University, United StatesReviewed by:
Kevin Y. Urayama, St. Luke’s International University, JapanMartha Jane Felini, University of North Texas Health Science Center, United States
Copyright © 2021 Marron, Brackmann, Kuhse, Christianson, Langner, Haug and Ahrens. This is an open-access article distributed under the terms of the Creative Commons Attribution License (CC BY). The use, distribution or reproduction in other forums is permitted, provided the original author(s) and the copyright owner(s) are credited and that the original publication in this journal is cited, in accordance with accepted academic practice. No use, distribution or reproduction is permitted which does not comply with these terms.
*Correspondence: Manuela Marron, sec-epi@leibniz-bips.de